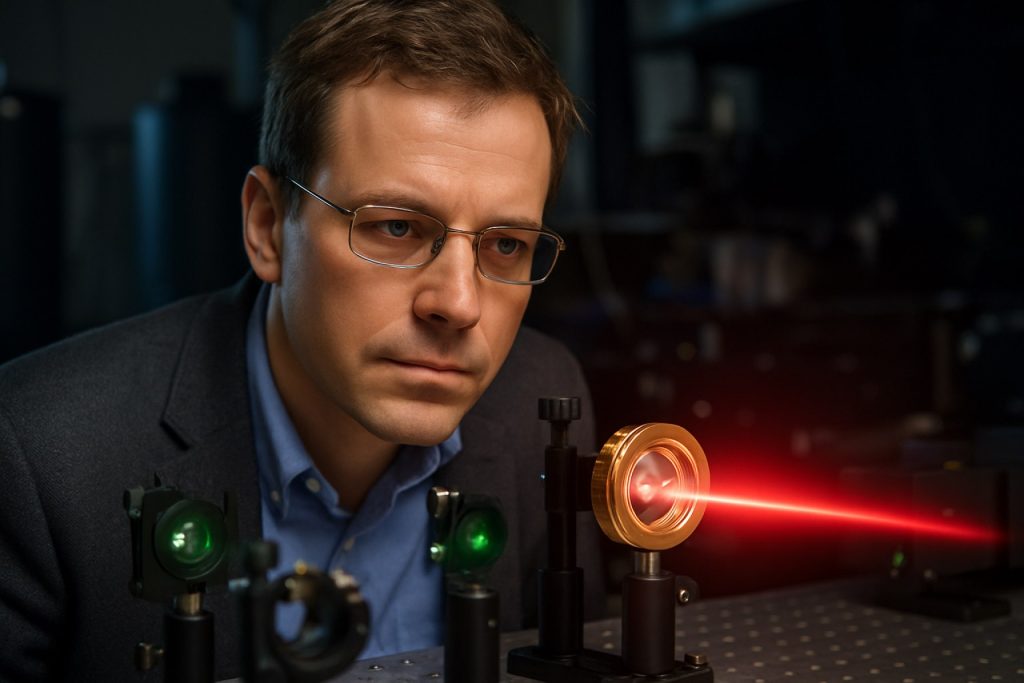
Zeptosecond Physics Explained: How Ultra-Fast Science is Redefining Our Understanding of Atomic and Subatomic Events. Discover the Breakthroughs and Future Impact of Zeptosecond Research. (2025)
- Introduction to Zeptosecond Physics: Defining the Zeptosecond Scale
- Historical Milestones: From Femtoseconds to Zeptoseconds
- Experimental Techniques: Capturing Zeptosecond Events
- Key Discoveries: Observing Electron Dynamics in Real Time
- Technological Innovations Enabling Zeptosecond Measurements
- Applications in Quantum Mechanics and Chemistry
- Leading Research Institutions and Collaborations
- Market and Public Interest: Growth Forecasts and Funding Trends
- Challenges and Limitations in Zeptosecond Research
- Future Outlook: Potential Breakthroughs and Societal Impact
- Sources & References
Introduction to Zeptosecond Physics: Defining the Zeptosecond Scale
Zeptosecond physics is an emerging frontier in ultrafast science, focusing on processes that occur on the timescale of a zeptosecond—one trillionth of a billionth of a second, or 10-21 seconds. This scale is three orders of magnitude shorter than the attosecond (10-18 seconds) regime, which itself only became experimentally accessible in the early 2000s. The zeptosecond domain is particularly significant for probing the most fundamental interactions in atomic and nuclear physics, such as the movement of electrons within atoms and the dynamics of nuclear reactions.
The first direct measurement of a process on the zeptosecond timescale was reported in 2020, when researchers observed the photoionization of a hydrogen molecule occurring in about 247 zeptoseconds. This milestone, achieved using advanced X-ray free-electron lasers and coincidence detection techniques, marked the beginning of experimental access to the zeptosecond regime. Since then, laboratories worldwide have been refining both the generation and measurement of zeptosecond-scale events, with a focus on improving temporal resolution and control over ultrafast photon sources.
The Max Planck Society and its associated institutes, such as the Max Planck Institute for Quantum Optics, have played a leading role in developing the theoretical framework and experimental tools necessary for zeptosecond physics. These efforts are complemented by large-scale facilities like the Deutsches Elektronen-Synchrotron (DESY) in Germany, which operates some of the world’s most advanced X-ray free-electron lasers. Such facilities are crucial for generating the high-energy, ultrashort pulses required to probe zeptosecond phenomena.
As of 2025, the field is rapidly advancing, with ongoing projects aimed at pushing the limits of temporal resolution even further. Researchers are now targeting the observation of electron dynamics within atomic nuclei and the direct measurement of quantum processes that were previously considered too fast to capture. The next few years are expected to see the development of even shorter light pulses, improved synchronization techniques, and new detection methods, all of which will expand the scope of zeptosecond physics.
The outlook for zeptosecond physics is highly promising. By enabling the observation and control of processes at the very heart of matter, this field is poised to deepen our understanding of quantum mechanics, nuclear structure, and fundamental interactions. The continued collaboration between leading research organizations and the deployment of next-generation light sources will be key drivers of progress in this ultrafast scientific domain.
Historical Milestones: From Femtoseconds to Zeptoseconds
The journey from femtosecond to zeptosecond physics marks a remarkable evolution in the ability to probe and manipulate ultrafast phenomena. The femtosecond (10-15 seconds) regime, pioneered in the late 20th century, enabled the observation of molecular vibrations and chemical reactions in real time. This era was notably advanced by the development of femtosecond lasers, a breakthrough recognized by the 1999 Nobel Prize in Chemistry awarded to Ahmed Zewail for his work on femtochemistry (Nobel Prize).
Progressing into the attosecond (10-18 seconds) domain, researchers achieved the generation of attosecond pulses in the early 2000s, allowing the direct observation of electron dynamics within atoms. This leap was facilitated by high-harmonic generation techniques and the refinement of ultrafast laser systems. The attosecond field matured rapidly, with significant contributions from institutions such as Max Planck Society and CERN, which have supported fundamental research in ultrafast science.
The transition to zeptosecond (10-21 seconds) physics represents the current frontier. In 2020, a landmark experiment led by researchers at the Max Planck Society and DESY (Deutsches Elektronen-Synchrotron) measured the photoemission delay of electrons from a hydrogen molecule, clocking the process at 247 zeptoseconds. This achievement set a new record for the shortest time interval ever measured and demonstrated the feasibility of observing electron motion on the scale of atomic nuclei.
As of 2025, zeptosecond physics is poised for further breakthroughs. Major research facilities, including DESY and CERN, are investing in next-generation X-ray free-electron lasers and advanced detection systems to push temporal resolution even further. These efforts aim to resolve fundamental questions about quantum tunneling, nuclear processes, and the interplay between electrons and nuclei. The European Synchrotron Radiation Facility and similar organizations are also expanding their capabilities to support zeptosecond-scale experiments.
Looking ahead, the next few years are expected to see the first direct measurements of zeptosecond-scale nuclear reactions and the refinement of theoretical models to interpret these ultrafast events. The continued collaboration between leading research organizations and the development of more powerful light sources will likely yield new insights into the fundamental mechanisms governing matter at its most elementary level.
Experimental Techniques: Capturing Zeptosecond Events
The pursuit of capturing and characterizing events on the zeptosecond (10-21 second) timescale has driven significant advances in experimental physics, particularly in ultrafast laser science and attosecond metrology. As of 2025, the field is marked by a convergence of high-intensity laser technology, advanced detection schemes, and international collaboration, enabling researchers to probe electron dynamics and fundamental quantum processes with unprecedented temporal resolution.
A cornerstone technique in zeptosecond physics is the use of high-harmonic generation (HHG) to produce ultrashort pulses of extreme ultraviolet (XUV) and X-ray light. These pulses, when synchronized with femtosecond or attosecond pump-probe setups, allow for the direct observation of electron motion within atoms and molecules. In 2020, a landmark experiment at the Max Planck Society’s Institute for Nuclear Physics demonstrated the measurement of photoionization in hydrogen molecules occurring within 247 zeptoseconds, setting a new benchmark for temporal resolution in atomic-scale processes.
Since then, facilities such as the Deutsches Elektronen-Synchrotron (DESY) and the Extreme Light Infrastructure (ELI) have expanded capabilities for generating and characterizing zeptosecond pulses. These organizations operate free-electron lasers (FELs) and petawatt-class laser systems, which are essential for producing the high photon energies and intensities required for zeptosecond-scale experiments. In 2023, DESY reported progress in compressing X-ray pulses to durations approaching the zeptosecond regime, leveraging advanced electron bunch shaping and synchronization techniques.
Detection and measurement at these timescales rely on streaking methods, where the timing of electron emission is mapped onto measurable energy shifts, and on coincidence spectroscopy, which correlates multiple particle emissions to reconstruct ultrafast dynamics. The integration of machine learning algorithms for data analysis is becoming increasingly important, as it enables the extraction of subtle temporal signatures from large, complex datasets generated by these experiments.
Looking ahead, the next few years are expected to see further reductions in pulse duration and improvements in timing precision, driven by upgrades at major research infrastructures and the development of novel laser architectures. The European Organization for Nuclear Research (CERN) and other global partners are exploring synergies between zeptosecond physics and high-energy particle physics, aiming to probe quantum electrodynamics and nuclear processes at their most fundamental level. These advances will not only deepen our understanding of ultrafast phenomena but may also open new avenues in quantum control and information science.
Key Discoveries: Observing Electron Dynamics in Real Time
The field of zeptosecond physics—where one zeptosecond equals 10-21 seconds—has rapidly advanced the ability to observe and control electron dynamics in real time. Since the first direct measurement of a process on the zeptosecond timescale in 2020, researchers have continued to push the boundaries of temporal resolution, enabling unprecedented insights into fundamental quantum processes.
A landmark achievement was reported in 2020, when a team at the Max Planck Society used attosecond and zeptosecond pulses to measure the photoionization of hydrogen molecules, capturing the time it takes for an electron to leave the atom. This experiment, which recorded a process lasting just 247 zeptoseconds, set a new benchmark for time-resolved measurements of electron motion. Since then, laboratories worldwide have refined these techniques, leveraging advances in ultrafast laser technology and synchronization methods.
By 2025, several research groups, including those at the European Organization for Nuclear Research (CERN) and the Deutsches Elektronen-Synchrotron (DESY), are developing next-generation light sources capable of generating even shorter pulses. These facilities are enabling the direct observation of electron correlation effects, charge migration, and quantum coherence in atoms and molecules. For example, DESY’s free-electron lasers are being used to probe the earliest steps of chemical reactions, capturing electron rearrangements on zeptosecond timescales.
Recent data from collaborative projects, such as those coordinated by the European Synchrotron Radiation Facility (ESRF), indicate that zeptosecond-resolved spectroscopy is now being applied to complex systems, including biomolecules and solid-state materials. These experiments are revealing how electronic excitations propagate and dissipate, with implications for quantum information science and ultrafast electronics.
Looking ahead, the outlook for zeptosecond physics is highly promising. Ongoing upgrades to major research infrastructures, such as the Extreme Light Infrastructure (ELI) and new beamlines at DESY and ESRF, are expected to further enhance temporal resolution and experimental flexibility. The next few years will likely see the first real-time movies of electron motion in increasingly complex environments, paving the way for breakthroughs in controlling chemical reactions, designing quantum devices, and understanding fundamental processes in matter at the shortest timescales accessible to science.
Technological Innovations Enabling Zeptosecond Measurements
Zeptosecond physics, the study of processes occurring on the timescale of 10-21 seconds, has rapidly advanced due to a series of technological innovations in ultrafast laser science and detection methods. As of 2025, several key breakthroughs are enabling researchers to probe atomic and subatomic events with unprecedented temporal resolution.
One of the most significant developments is the refinement of attosecond pulse generation, which forms the foundation for reaching zeptosecond regimes. High-harmonic generation (HHG) in gases, driven by intense femtosecond lasers, has been optimized to produce ever-shorter pulses. Recent advances in phase-matching techniques and the use of mid-infrared driving lasers have allowed the extension of attosecond pulses toward the zeptosecond domain. Laboratories affiliated with major research organizations, such as Max Planck Society and Centre National de la Recherche Scientifique (CNRS), have reported the generation of isolated pulses with durations approaching a few hundred zeptoseconds, enabling the direct observation of electron dynamics within atoms.
Another critical innovation is the development of streaking and pump-probe techniques with zeptosecond precision. By synchronizing X-ray free-electron lasers (XFELs) with optical lasers, researchers can now resolve electron emission and charge migration on zeptosecond timescales. Facilities such as the Deutsches Elektronen-Synchrotron (DESY) and Paul Scherrer Institute have upgraded their XFEL infrastructures to support these ultrafast experiments, providing higher photon energies and improved timing synchronization.
Detection technology has also seen substantial progress. The latest generation of time-of-flight spectrometers and electron detectors, featuring sub-femtosecond response times and enhanced quantum efficiency, are now standard in leading laboratories. These instruments are crucial for capturing the fleeting signatures of zeptosecond-scale events, such as Auger decay and photoionization delays.
Looking ahead, the next few years are expected to bring further miniaturization and integration of laser and detection systems, making zeptosecond measurement capabilities more accessible to a broader range of research institutions. International collaborations, such as those coordinated by CERN and the American Physical Society, are fostering the development of standardized protocols and data-sharing platforms to accelerate discoveries in zeptosecond physics. As these technologies mature, they are poised to unlock new insights into quantum dynamics, chemical reactions, and fundamental interactions at the smallest temporal scales.
Applications in Quantum Mechanics and Chemistry
Zeptosecond physics, the study of processes occurring on the timescale of 10-21 seconds, is rapidly transforming our understanding of quantum mechanics and chemistry. Since the first direct measurement of a zeptosecond event in 2020, researchers have been leveraging this ultrafast temporal resolution to probe the most fundamental quantum phenomena, particularly those involving electron dynamics within atoms and molecules.
In 2025, the field is witnessing a surge in experimental capabilities, driven by advances in attosecond and zeptosecond laser pulse generation. Facilities such as the Max Planck Society’s institutes and the Deutsches Elektronen-Synchrotron (DESY) are at the forefront, utilizing free-electron lasers and high-harmonic generation sources to create and measure zeptosecond-scale events. These tools allow scientists to observe electron tunneling, charge migration, and the initial steps of chemical bond formation and breaking with unprecedented clarity.
One of the most significant applications in quantum mechanics is the direct observation of electron correlation effects and quantum coherence. Zeptosecond pulses enable the tracking of entangled electron pairs and the measurement of decoherence timescales, which are critical for quantum information science. In 2025, collaborative projects between European and Asian research consortia are focusing on using zeptosecond metrology to benchmark quantum simulation algorithms and validate quantum computing hardware at the level of fundamental electron motion.
In chemistry, zeptosecond physics is opening new avenues for controlling and steering chemical reactions. By timing laser pulses to coincide with specific electron transitions, researchers can influence reaction pathways, potentially leading to the development of ultrafast photochemistry and new catalytic processes. The French National Centre for Scientific Research (CNRS) and the RIKEN institute in Japan are leading efforts to apply zeptosecond spectroscopy to complex organic molecules, aiming to map out the earliest quantum steps in photosynthesis and vision.
Looking ahead, the next few years are expected to bring further integration of zeptosecond techniques with electron microscopy and X-ray diffraction, enabling real-time imaging of quantum processes in both isolated molecules and condensed matter systems. As international collaborations expand and laser technology matures, zeptosecond physics is poised to become a cornerstone of quantum science, with transformative impacts on quantum computing, molecular engineering, and our fundamental understanding of matter.
Leading Research Institutions and Collaborations
Zeptosecond physics, the study of processes occurring on the timescale of 10-21 seconds, has rapidly advanced due to the concerted efforts of leading research institutions and international collaborations. As of 2025, several organizations are at the forefront, driving both fundamental discoveries and technological innovations in ultrafast science.
A central role is played by the Max Planck Society, particularly through its Max Planck Institute of Quantum Optics (MPQ) in Germany. MPQ researchers were instrumental in the first direct measurement of zeptosecond-scale events, such as the 2020 observation of photoionization delays in helium atoms. Their ongoing work leverages attosecond and zeptosecond laser pulses to probe electron dynamics, with new experiments planned to resolve even shorter timescales and more complex atomic systems.
In France, the Centre National de la Recherche Scientifique (CNRS) coordinates several national and European projects focused on ultrafast phenomena. CNRS laboratories, often in partnership with universities and synchrotron facilities, are developing next-generation light sources and detection techniques to push the temporal resolution boundary further into the zeptosecond regime.
The Deutsches Elektronen-Synchrotron (DESY) in Germany is another major player, operating large-scale X-ray free-electron lasers (XFELs) that enable the generation of ultrashort pulses. DESY’s collaborations with European XFEL and other international partners are crucial for experiments that require both high photon energies and extreme temporal precision. These facilities are expected to support a new wave of zeptosecond-scale studies in the coming years.
In the United States, the Los Alamos National Laboratory and the Lawrence Livermore National Laboratory are investing in ultrafast science, with research programs targeting the control and measurement of electron motion at zeptosecond timescales. These labs often collaborate with academic institutions and international consortia to share expertise and infrastructure.
Looking ahead, the field is marked by increasing global collaboration. Initiatives such as the Extreme Light Infrastructure (ELI), a pan-European project, are bringing together scientists from across the continent to develop the world’s most advanced laser systems. ELI’s facilities in the Czech Republic, Hungary, and Romania are expected to become operational for zeptosecond research by the mid-2020s, providing unprecedented capabilities for probing matter at its most fundamental level.
Overall, the synergy between leading research institutions, large-scale facilities, and international collaborations is accelerating progress in zeptosecond physics. The next few years are poised to deliver breakthroughs in our understanding of ultrafast processes, with implications for quantum technologies, materials science, and fundamental physics.
Market and Public Interest: Growth Forecasts and Funding Trends
Zeptosecond physics, the study of phenomena occurring on the timescale of 10-21 seconds, has rapidly transitioned from a niche research area to a focal point of global scientific investment. As of 2025, the field is experiencing a surge in both public and private funding, driven by its potential to revolutionize ultrafast science, quantum technologies, and materials research.
Major research organizations and government agencies are at the forefront of this momentum. The Max Planck Society in Germany, renowned for its leadership in fundamental physics, continues to expand its attosecond and zeptosecond research programs, particularly at the Max Planck Institute of Quantum Optics. Similarly, the Centre National de la Recherche Scientifique (CNRS) in France and the Paul Scherrer Institute in Switzerland are investing in next-generation light sources and ultrafast laser facilities, aiming to probe electron dynamics at zeptosecond resolution.
In the United States, the U.S. Department of Energy (DOE) has increased funding for ultrafast science through its Office of Science, supporting projects at national laboratories such as SLAC and Argonne. These investments are reflected in the construction and upgrade of X-ray free-electron lasers (XFELs), which are essential for generating the ultrashort pulses required for zeptosecond experiments. The DOE’s 2024–2026 budget outlines continued prioritization of quantum and ultrafast science, with specific calls for proposals in zeptosecond-scale measurement and control.
On the commercial side, companies specializing in laser technology and photonics, such as TRUMPF and Thorlabs, are reporting increased demand for components capable of supporting zeptosecond research. These firms are collaborating with academic and government labs to develop next-generation pulse compression and detection systems, anticipating a growing market for ultrafast measurement tools in both research and industry.
Forecasts for the next few years suggest that the global market for ultrafast lasers and related instrumentation will grow at a double-digit annual rate, with zeptosecond physics representing a key driver. Public interest is also rising, as breakthroughs in this field promise advances in quantum computing, medical imaging, and materials science. Funding agencies in Asia, notably the RIKEN institute in Japan and the National Natural Science Foundation of China, are launching new grant programs to support zeptosecond-scale research, further intensifying international competition and collaboration.
Overall, the outlook for zeptosecond physics in 2025 and beyond is marked by robust funding, expanding infrastructure, and a growing ecosystem of public and private stakeholders. This dynamic environment is expected to accelerate both fundamental discoveries and the commercialization of ultrafast technologies in the coming years.
Challenges and Limitations in Zeptosecond Research
Zeptosecond physics, which investigates processes occurring on the timescale of 10-21 seconds, stands at the frontier of ultrafast science. Despite remarkable progress, several challenges and limitations continue to shape the field as of 2025 and are likely to persist in the near future.
A primary challenge is the generation and precise measurement of zeptosecond pulses. Current state-of-the-art techniques, such as attosecond streaking and high-harmonic generation, have only recently approached the zeptosecond regime. Achieving reliable, reproducible zeptosecond pulses requires further advances in laser technology, phase control, and synchronization. The complexity of these experimental setups, often involving large-scale facilities like free-electron lasers, limits widespread access and scalability. For example, facilities such as those operated by Deutsches Elektronen-Synchrotron (DESY) and Paul Scherrer Institute are at the forefront, but their resources are finite and highly competitive.
Another significant limitation is the detection and interpretation of zeptosecond-scale events. The ultrashort duration of these phenomena means that conventional detectors and electronics are too slow to resolve them directly. Researchers rely on indirect methods, such as pump-probe spectroscopy, which require sophisticated data analysis and modeling. This introduces uncertainties and potential ambiguities in interpreting results, especially when probing complex quantum systems or electron dynamics in atoms and molecules.
Material and technological constraints also play a role. The intense fields required for zeptosecond experiments can damage optical components and targets, necessitating the development of more robust materials and innovative engineering solutions. Additionally, the synchronization of multiple laser systems to within zeptosecond precision remains a formidable technical hurdle.
On the theoretical side, modeling electron and nuclear dynamics at zeptosecond timescales demands significant computational resources and the development of new quantum mechanical frameworks. The interplay between theory and experiment is crucial, but discrepancies between predictions and measurements can arise due to the extreme conditions and the limits of current models.
Looking ahead, the field anticipates incremental progress as new laser sources, detection schemes, and computational methods are developed. International collaborations, such as those coordinated by CERN and MAX IV Laboratory, are expected to play a pivotal role in overcoming these barriers. However, the high cost, technical complexity, and need for interdisciplinary expertise will likely continue to constrain the pace and breadth of zeptosecond research through the next few years.
Future Outlook: Potential Breakthroughs and Societal Impact
Zeptosecond physics, the study of phenomena occurring on the timescale of 10-21 seconds, stands at the frontier of ultrafast science. As of 2025, the field is poised for transformative breakthroughs, driven by rapid advances in laser technology, detection methods, and theoretical modeling. The ability to observe and manipulate processes at the zeptosecond scale promises to deepen our understanding of fundamental quantum dynamics and could catalyze innovations across multiple scientific and technological domains.
Recent years have seen landmark achievements, such as the first direct measurement of photoionization delays in atoms on the zeptosecond timescale, accomplished by international collaborations using attosecond pulse generation and streaking techniques. These experiments, often conducted at large-scale facilities like the Deutsches Elektronen-Synchrotron (DESY) and the European Organization for Nuclear Research (CERN), have demonstrated the feasibility of probing electron dynamics with unprecedented temporal resolution. In 2023 and 2024, research teams refined these methods, achieving sub-100 zeptosecond temporal precision in tracking electron ejection and correlation effects in complex atoms and molecules.
Looking ahead to 2025 and beyond, several key directions are anticipated:
- Enhanced Laser Sources: The development of next-generation free-electron lasers and high-harmonic generation sources is expected to deliver even shorter and more intense pulses, enabling routine access to zeptosecond regimes. Facilities such as European XFEL are investing in upgrades to support these capabilities.
- Quantum Control: Researchers aim to not only observe but also control electron motion on zeptosecond timescales, potentially steering chemical reactions or quantum information processes at their most fundamental level.
- New States of Matter: Zeptosecond techniques may allow the creation and study of exotic states, such as electron-hole plasmas or transient quantum phases, with implications for condensed matter physics and materials science.
- Particle Physics Synergy: Collaborations with high-energy physics institutions, including CERN, are exploring how zeptosecond pulses can probe subatomic processes, such as quark-gluon dynamics, with temporal resolution matching the natural timescales of strong-force interactions.
The societal impact of zeptosecond physics could be profound. In the near term, advances in ultrafast imaging and spectroscopy will enhance our ability to design novel materials, optimize chemical processes, and develop new quantum technologies. In the longer term, the fundamental insights gained may inform next-generation electronics, secure communications, and even medical diagnostics. As international research organizations and consortia continue to invest in this field, zeptosecond physics is set to remain a vibrant and rapidly evolving area of science through 2025 and the years ahead.
Sources & References
- Max Planck Society
- Deutsches Elektronen-Synchrotron (DESY)
- Nobel Prize
- CERN
- European Synchrotron Radiation Facility
- Extreme Light Infrastructure (ELI)
- Centre National de la Recherche Scientifique (CNRS)
- Paul Scherrer Institute
- CERN
- RIKEN
- Los Alamos National Laboratory
- Lawrence Livermore National Laboratory
- Max Planck Society
- TRUMPF
- Thorlabs
- MAX IV Laboratory
- European XFEL