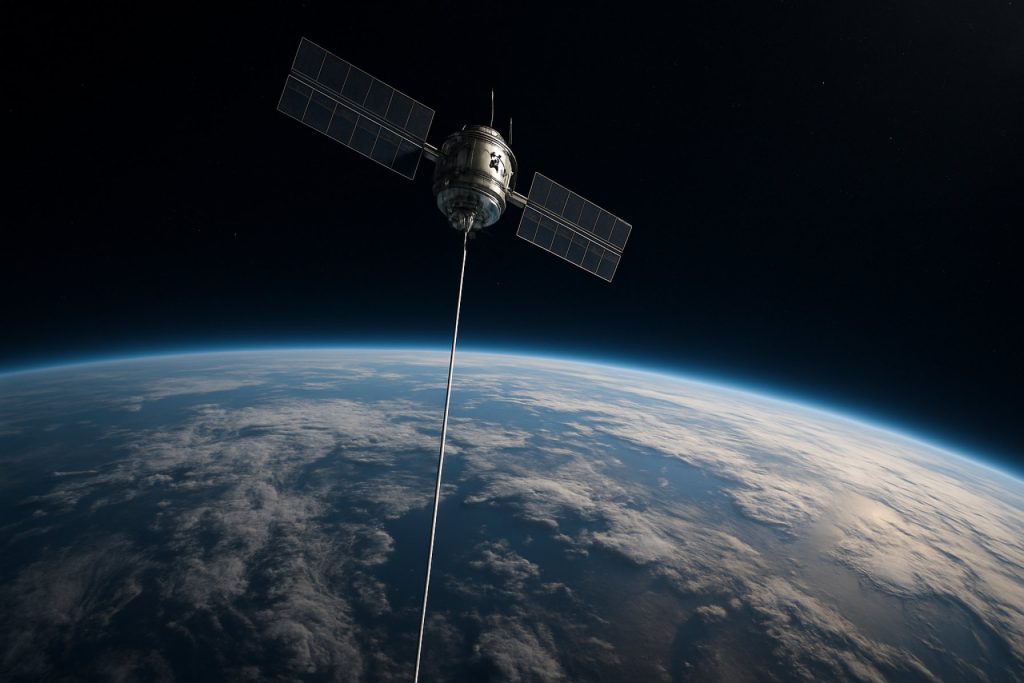
Unlocking the Future of Space Operations: How Geosynchronous Orbit Electrodynamic Tether Systems Could Transform Satellite Lifespans and Space Debris Management. Explore the Science, Technology, and Potential of This Game-Changing Innovation. (2025)
- Introduction to Geosynchronous Orbit Electrodynamic Tether Systems
- Historical Development and Key Milestones
- Core Principles: Physics and Engineering of Electrodynamic Tethers
- Current Applications in Geosynchronous Orbit
- Major Projects and Demonstrations (e.g., NASA, JAXA Initiatives)
- Advantages Over Conventional Propulsion and Debris Mitigation Methods
- Technical Challenges and Limitations
- Market and Public Interest Forecast: Growth Potential and Adoption Rates
- Regulatory, Safety, and Policy Considerations
- Future Outlook: Innovations, Research Directions, and Long-Term Impact
- Sources & References
Introduction to Geosynchronous Orbit Electrodynamic Tether Systems
Geosynchronous Orbit Electrodynamic Tether Systems (GO-EDTS) represent a promising class of space technologies designed to provide propulsion, power generation, and orbital maneuvering capabilities for satellites and spacecraft operating in geosynchronous orbit (GEO). A geosynchronous orbit is a circular orbit around Earth with an orbital period matching the planet’s rotation, allowing satellites to remain fixed relative to a point on the equator. This unique orbital regime is critical for telecommunications, weather monitoring, and surveillance applications, making efficient station-keeping and end-of-life disposal methods highly valuable.
An electrodynamic tether is a long, conductive wire or tape deployed from a spacecraft, which interacts with Earth’s magnetic field to generate electrical current and, consequently, a Lorentz force. This force can be harnessed for propulsion or to alter the spacecraft’s orbit without the need for conventional propellant. The underlying principle relies on the motion of the tether through the geomagnetic field, inducing a voltage along its length. By controlling the direction and magnitude of the current, the system can either raise or lower the spacecraft’s orbit, or generate electrical power for onboard systems.
The application of electrodynamic tethers in geosynchronous orbit presents unique challenges and opportunities. Unlike low Earth orbit (LEO), where the geomagnetic field is stronger and plasma density is higher, GEO features a weaker magnetic field and lower plasma density, which can reduce the efficiency of current collection and force generation. Nevertheless, the potential benefits—such as propellantless station-keeping, debris mitigation, and end-of-life deorbiting—have driven ongoing research and development efforts by leading space agencies and organizations.
Notably, NASA has conducted extensive studies and technology demonstrations related to electrodynamic tethers, including the Tethered Satellite System (TSS) missions and ongoing theoretical work on advanced tether concepts. The European Space Agency (ESA) has also explored tether-based solutions for space debris removal and satellite servicing. In addition, organizations such as the Japan Aerospace Exploration Agency (JAXA) have tested electrodynamic tethers in orbit, most notably with the Kounotori Integrated Tether Experiment (KITE) mission.
As of 2025, geosynchronous orbit electrodynamic tether systems remain an area of active research, with ongoing efforts to address technical challenges related to tether materials, current collection efficiency, and long-term reliability in the harsh GEO environment. The successful deployment of GO-EDTS could significantly enhance the sustainability and operational flexibility of geosynchronous satellites, supporting the growing demands of global communications and Earth observation infrastructure.
Historical Development and Key Milestones
The concept of electrodynamic tethers (EDTs) for space applications dates back to the 1960s, when researchers first theorized that long, conductive wires deployed in orbit could interact with Earth’s magnetic field to generate electrical power or propulsion. Early studies were primarily focused on low Earth orbit (LEO), where the geomagnetic field is stronger and plasma density is higher, making electrodynamic effects more pronounced. However, as the need for innovative propulsion and power solutions in higher orbits grew, attention gradually shifted toward the potential of EDTs in geosynchronous orbit (GEO).
A significant milestone occurred in the 1980s, when the National Aeronautics and Space Administration (NASA) and the Italian Space Agency (ASI) collaborated on the Tethered Satellite System (TSS) missions. While these missions were conducted in LEO, they provided critical insights into tether deployment dynamics, current collection, and plasma interactions—laying the groundwork for future GEO applications. The TSS-1 (1992) and TSS-1R (1996) missions demonstrated both the promise and the technical challenges of tether systems, such as deployment control and survivability in the harsh space environment.
By the late 1990s and early 2000s, theoretical and simulation studies began to address the unique challenges of operating EDTs in GEO, where the magnetic field is weaker and plasma conditions differ significantly from LEO. Researchers at institutions such as the NASA Glenn Research Center and the European Space Agency (ESA) explored the feasibility of using long tethers for station-keeping, end-of-life deorbiting, and even power generation for satellites in geosynchronous orbit. These studies identified key technical hurdles, including efficient current collection in low-density plasma and the need for robust materials to withstand micrometeoroid impacts and space weather effects.
A pivotal development in the 2010s was the advancement of high-conductivity tether materials and autonomous deployment mechanisms, which made the concept of GEO EDTs more practical. The Japan Aerospace Exploration Agency (JAXA) and private sector partners began investigating tether-based solutions for space debris mitigation and satellite servicing in GEO, reflecting a growing international interest in the technology.
By 2025, geosynchronous orbit electrodynamic tether systems have progressed from theoretical constructs to the verge of in-orbit demonstration. Ongoing research and technology maturation, led by agencies such as NASA, ESA, and JAXA, continue to address the remaining challenges, with the goal of enabling sustainable, propellantless propulsion and power solutions for the next generation of GEO satellites and infrastructure.
Core Principles: Physics and Engineering of Electrodynamic Tethers
Electrodynamic tether (EDT) systems are long, conductive wires deployed from spacecraft to interact with planetary magnetic fields, generating electrical currents and forces through fundamental electromagnetic principles. In the context of geosynchronous orbit (GEO)—an altitude of approximately 35,786 km where satellites match Earth’s rotation—EDT systems present unique physics and engineering challenges and opportunities.
The core principle underlying EDTs is the Lorentz force: when a conductive tether moves through a magnetic field, such as Earth’s, it experiences a force perpendicular to both its velocity and the magnetic field direction. This motion induces an electromotive force (EMF) along the tether, driving a current if the circuit is closed, either through the ambient plasma or via onboard systems. The resulting current interacts with the geomagnetic field, producing a force that can be harnessed for propulsion, station-keeping, or deorbiting without expending propellant.
In low Earth orbit (LEO), EDTs have been demonstrated to generate significant thrust or drag due to the relatively strong geomagnetic field and dense ionospheric plasma. However, at GEO, the situation is markedly different. The Earth’s magnetic field is much weaker, and the plasma density is orders of magnitude lower. This reduces both the induced EMF and the efficiency of current collection, presenting a major engineering challenge for tether design and operation. To compensate, GEO EDT systems require longer tethers—potentially tens of kilometers—and advanced materials with high conductivity and low mass, such as aluminum or advanced composites. The tether must also be engineered to withstand the harsh space environment, including micrometeoroid impacts, thermal cycling, and radiation.
A critical engineering consideration is the method of current collection and emission. At GEO, the low plasma density makes traditional bare tether designs less effective. Instead, systems may employ electron emitters, such as hollow cathodes, to close the electrical circuit. Power management and control electronics must be robust, as the induced voltages can reach several kilovolts, and the system must safely dissipate or utilize the generated electrical energy.
The potential applications of GEO EDT systems include propellantless station-keeping, end-of-life deorbiting, and even power generation for onboard systems. These capabilities align with the growing need for sustainable space operations and debris mitigation in the increasingly crowded GEO belt. Research and development in this field are supported by organizations such as NASA and the European Space Agency, both of which have conducted studies and technology demonstrations of EDTs in various orbital regimes.
In summary, the physics and engineering of geosynchronous orbit electrodynamic tether systems are governed by the interplay of electromagnetic induction, plasma physics, and advanced materials science. Overcoming the unique challenges of the GEO environment is essential for realizing the full potential of EDTs in future space infrastructure.
Current Applications in Geosynchronous Orbit
Electrodynamic tether (EDT) systems have long been proposed as a means of propulsion, power generation, and orbital maneuvering in space. In geosynchronous orbit (GEO), these systems are of particular interest due to the unique challenges and opportunities presented by the high-altitude, stable orbital environment. As of 2025, the practical application of EDTs in GEO remains largely experimental, but several initiatives and research programs are actively exploring their potential.
The primary appeal of EDTs in GEO lies in their ability to generate thrust or drag without the need for propellant, by interacting with the Earth’s magnetic field and ionospheric plasma. This capability is especially valuable for station-keeping, end-of-life deorbiting, and potentially for power generation. However, the relatively weak magnetic field at GEO altitudes, compared to low Earth orbit (LEO), presents significant engineering challenges. Despite these hurdles, organizations such as the National Aeronautics and Space Administration (NASA) and the European Space Agency (ESA) have conducted studies and small-scale experiments to assess the feasibility of EDTs in GEO.
One notable application under investigation is the use of EDTs for end-of-life disposal of GEO satellites. Traditional chemical propulsion methods for moving defunct satellites to graveyard orbits require significant fuel reserves, which can limit operational lifespans. EDTs offer a propellantless alternative, potentially extending mission durations and reducing costs. The European Space Agency has supported research into tether-based deorbiting and re-orbiting systems, including the development of advanced materials and deployment mechanisms suitable for the GEO environment.
In addition to deorbiting, EDTs in GEO are being considered for power generation. By harnessing the motional electromotive force as the tether moves through the geomagnetic field, it is theoretically possible to generate electrical power for satellite systems. While the power output at GEO is lower than in LEO, ongoing research aims to optimize tether length, orientation, and material conductivity to maximize efficiency. NASA’s ongoing technology roadmaps include the study of EDTs as part of broader efforts to develop sustainable, in-space power and propulsion solutions.
Although no large-scale operational EDT system has yet been deployed in GEO as of 2025, the continued interest and investment by major space agencies and research institutions underscore the potential of this technology. As advancements in materials science and space engineering progress, EDTs may soon transition from experimental concepts to practical tools for GEO satellite management and sustainability.
Major Projects and Demonstrations (e.g., NASA, JAXA Initiatives)
Geosynchronous orbit electrodynamic tether (EDT) systems have attracted significant attention as a means to provide propellantless propulsion, station-keeping, and deorbiting capabilities for satellites and space debris in the geosynchronous belt. Several major space agencies and organizations have initiated projects and demonstrations to advance the technology readiness of EDTs for geosynchronous applications.
The National Aeronautics and Space Administration (NASA) has been a pioneer in electrodynamic tether research, with a history of both low Earth orbit (LEO) and higher altitude tether experiments. While most early demonstrations, such as the Tethered Satellite System (TSS) missions, focused on LEO, NASA has since expanded its research to address the unique challenges of geosynchronous orbit (GEO). In recent years, NASA’s Marshall Space Flight Center has led studies on the feasibility of long-duration EDT operations in GEO, including the development of advanced tether materials and power management systems suitable for the high-radiation and micrometeoroid environment of this orbital regime. These efforts are part of NASA’s broader goal to enable sustainable satellite operations and debris mitigation in GEO.
The Japan Aerospace Exploration Agency (JAXA) has also played a leading role in EDT technology, particularly through its Kounotori Integrated Tether Experiment (KITE) and the earlier Tether Experiment (T-Rex). While these missions were conducted in LEO, JAXA has published conceptual studies and technology roadmaps for extending EDT applications to geosynchronous orbit. JAXA’s research emphasizes the use of EDTs for end-of-life deorbiting of GEO satellites, which is critical for maintaining the long-term sustainability of this valuable orbital region. The agency collaborates with Japanese industry partners to develop robust tether deployment mechanisms and autonomous control systems that could be adapted for GEO missions.
In Europe, the European Space Agency (ESA) has supported several studies and technology development projects related to electrodynamic tethers, including the Electrodynamic Tether Technology for Passive Satellite Deorbiting (EDT4PASS) initiative. While ESA’s primary focus has been on LEO applications, the agency’s Clean Space initiative has identified GEO debris mitigation as a future target for EDT deployment. ESA’s work includes the development of simulation tools and ground-based experiments to validate tether dynamics and current collection in the GEO environment.
Collectively, these major projects and demonstrations by NASA, JAXA, and ESA are laying the groundwork for future in-orbit demonstrations of electrodynamic tether systems in geosynchronous orbit. Their efforts are crucial for addressing the technical, operational, and regulatory challenges associated with deploying and operating EDTs in this strategically important region of space.
Advantages Over Conventional Propulsion and Debris Mitigation Methods
Geosynchronous Orbit Electrodynamic Tether (EDT) systems present several significant advantages over conventional propulsion and debris mitigation methods, particularly in the context of long-term sustainability and operational efficiency in geosynchronous orbit (GEO). Unlike traditional chemical or electric propulsion, EDTs utilize the interaction between a conductive tether and the Earth’s magnetic field to generate thrust or drag without expending propellant. This fundamental difference offers a range of benefits for both station-keeping and end-of-life deorbiting of satellites.
One of the primary advantages of EDT systems is their propellantless operation. Conventional propulsion systems require substantial onboard fuel reserves, which add mass and limit operational lifetimes. In contrast, EDTs draw electrical current from the ambient plasma and Earth’s magnetic field, enabling continuous thrust or drag as long as the tether remains functional. This can significantly extend mission durations and reduce launch mass requirements, leading to cost savings and increased payload capacity for satellite operators.
In terms of debris mitigation, EDTs offer a unique capability for controlled deorbiting of defunct satellites and upper stages. Traditional methods often rely on residual propellant or mechanical devices, which may fail or be depleted before end-of-life. EDTs, however, can provide reliable, autonomous deorbiting by generating Lorentz forces that gradually lower the satellite’s orbit, even after primary mission completion. This approach aligns with international guidelines for space debris mitigation and supports the long-term sustainability of the GEO environment.
Additionally, EDT systems can be designed for dual-use: providing both station-keeping during operational life and deorbiting at end-of-life, further enhancing their value proposition. The ability to perform these functions without propellant not only reduces operational complexity but also minimizes the risk of creating additional debris through propulsion system failures or explosions.
- Reduced Operational Costs: By eliminating the need for large propellant reserves, EDTs lower launch and operational expenses.
- Extended Satellite Lifespan: Continuous, propellantless thrust enables longer mission durations and more flexible station-keeping.
- Enhanced Debris Mitigation: Reliable, autonomous deorbiting capabilities help prevent the accumulation of space debris in GEO.
- Environmental Sustainability: EDTs support compliance with international debris mitigation standards, contributing to the preservation of the orbital environment.
Research and demonstration missions by organizations such as the National Aeronautics and Space Administration and the European Space Agency have validated the fundamental principles of electrodynamic tethers, and ongoing development aims to scale these systems for operational use in GEO. As the demand for sustainable space operations grows, EDTs are poised to play a critical role in the future of satellite propulsion and debris management.
Technical Challenges and Limitations
Geosynchronous Orbit (GEO) Electrodynamic Tether (EDT) systems present a promising avenue for propellantless propulsion, station-keeping, and deorbiting in the GEO regime. However, their practical implementation faces several significant technical challenges and limitations that must be addressed for operational viability.
One of the foremost challenges is the weakness of the geomagnetic field at GEO altitudes. The effectiveness of EDTs relies on the interaction between the tether and the Earth’s magnetic field to generate Lorentz forces. At GEO, approximately 35,786 km above Earth’s surface, the geomagnetic field strength is less than 0.1% of its value at low Earth orbit (LEO), drastically reducing the available force for tether-based propulsion or drag. This limitation necessitates either extremely long tethers—potentially tens to hundreds of kilometers—or advanced materials and current collection techniques to achieve meaningful thrust or drag, which introduces further engineering complexities (NASA).
Material and structural challenges are also pronounced. Tethers must be lightweight yet capable of withstanding micrometeoroid and orbital debris impacts, as well as the harsh radiation environment of GEO. The long tether lengths required exacerbate vulnerability to damage and increase the risk of tether breakage or entanglement. Advanced materials such as high-strength polymers or carbon nanotube composites are under investigation, but their large-scale deployment in space remains unproven (European Space Agency).
Current collection and emission at GEO is another technical hurdle. Efficient electron collection and emission are essential for closing the electrical circuit in EDT systems. However, the low plasma density at GEO makes it difficult to collect and emit sufficient current, reducing system efficiency. Innovative solutions, such as plasma contactors or electron guns, are being explored, but these add complexity, mass, and power requirements to the system (Japan Aerospace Exploration Agency).
Attitude and orbital control present further complications. The deployment and stabilization of long tethers in GEO require precise control to avoid oscillations, unwanted rotations, or collisions with other satellites. The dynamic environment, including gravitational perturbations and solar radiation pressure, can induce tether libration or instability, complicating station-keeping and maneuvering operations.
Finally, regulatory and operational limitations must be considered. The deployment of long tethers in the crowded GEO belt raises concerns about collision risk and space debris generation, necessitating robust tracking, coordination, and end-of-life disposal strategies in compliance with international guidelines (United Nations Office for Outer Space Affairs).
In summary, while GEO EDT systems offer unique advantages, overcoming the technical and operational challenges outlined above is essential for their successful adoption in future space missions.
Market and Public Interest Forecast: Growth Potential and Adoption Rates
The market and public interest in Geosynchronous Orbit Electrodynamic Tether (EDT) Systems are poised for significant growth in 2025, driven by the increasing demand for sustainable and cost-effective space operations. EDT systems, which utilize long, conductive tethers to generate thrust or drag via interaction with the Earth’s magnetic field, offer a propellantless alternative for station-keeping, orbital maneuvering, and end-of-life deorbiting of satellites in geosynchronous orbit (GEO). This technology aligns with the global push for greener space solutions and the need to address the growing issue of space debris.
Key space agencies and organizations, such as the National Aeronautics and Space Administration (NASA) and the European Space Agency (ESA), have demonstrated ongoing interest in EDT research and technology demonstration missions. NASA’s past and current tether experiments, including the Tethered Satellite System and the ProSEDS project, have laid the groundwork for future commercial adoption. ESA, through its Clean Space initiative, has also explored EDTs as a viable method for satellite deorbiting and debris mitigation, reflecting a broader institutional commitment to sustainable space operations.
The commercial satellite sector is a primary driver of market growth. With over 400 operational satellites in GEO and a steady increase in satellite launches projected for telecommunications, Earth observation, and defense, the need for efficient station-keeping and end-of-life solutions is acute. EDT systems promise to reduce operational costs by minimizing fuel requirements and extending satellite lifespans, making them attractive to satellite operators and manufacturers. Companies specializing in advanced space propulsion and debris mitigation, such as those collaborating with major agencies, are expected to accelerate technology maturation and deployment.
Public interest is also rising, fueled by heightened awareness of space sustainability and the risks posed by orbital debris. International regulatory bodies, including the United Nations Office for Outer Space Affairs (UNOOSA), are increasingly advocating for responsible end-of-life practices, which may soon mandate the adoption of technologies like EDTs for GEO satellites. This regulatory momentum, combined with the potential for cost savings and environmental benefits, is expected to drive adoption rates upward.
In summary, 2025 is likely to see a marked increase in both market activity and public support for Geosynchronous Orbit Electrodynamic Tether Systems. As technology demonstrations transition to operational deployments, and as regulatory and commercial pressures mount, EDTs are positioned to become a standard component of GEO satellite design and operations.
Regulatory, Safety, and Policy Considerations
The deployment and operation of electrodynamic tether (EDT) systems in geosynchronous orbit (GEO) present a unique set of regulatory, safety, and policy challenges. As these systems interact directly with the Earth’s magnetosphere and ionosphere to generate thrust or electrical power, their use in GEO—a region densely populated with critical communications, weather, and navigation satellites—necessitates careful oversight and coordination.
From a regulatory perspective, the use of EDTs in GEO falls under the purview of several international and national frameworks. The United Nations Office for Outer Space Affairs (UNOOSA) provides the foundational legal structure through treaties such as the Outer Space Treaty (OST) and the Registration Convention, which require states to authorize and continually supervise space activities, including those involving novel propulsion technologies. Additionally, the International Telecommunication Union (ITU) manages the allocation of GEO slots and radio frequencies, which may be affected by the electromagnetic emissions or potential interference from EDT operations.
National regulatory bodies, such as the Federal Communications Commission (FCC) in the United States and the European Space Agency (ESA) in Europe, also play significant roles in licensing and oversight. These agencies assess the potential for radiofrequency interference, compliance with debris mitigation guidelines, and adherence to national security requirements. The increasing interest in EDTs for end-of-life satellite deorbiting or station-keeping in GEO has prompted these organizations to consider updating or clarifying existing regulations to address the unique operational profiles and risks associated with tether systems.
Safety considerations are paramount, particularly regarding the risk of tether breakage, uncontrolled re-entry, or inadvertent collisions with other GEO assets. The long, conductive nature of EDTs increases the probability of entanglement or fragmentation, which could exacerbate the space debris problem. As a result, mission proposals involving EDTs are subject to rigorous risk assessments and may require the implementation of fail-safe mechanisms, real-time monitoring, and contingency plans for rapid tether retraction or jettisoning.
Policy discussions are also evolving to address the dual-use nature of EDT technology, which could have both civilian and military applications. Transparency, data sharing, and international cooperation are increasingly emphasized to build trust and prevent misunderstandings. As the technology matures, ongoing dialogue among stakeholders—including satellite operators, regulatory agencies, and international organizations—will be essential to ensure that EDT deployment in GEO enhances sustainability and safety without compromising the operational integrity of this vital orbital regime.
Future Outlook: Innovations, Research Directions, and Long-Term Impact
The future of geosynchronous orbit electrodynamic tether (EDT) systems is poised for significant advancements, driven by ongoing innovations in materials science, power management, and orbital mechanics. As of 2025, research is intensifying on the development of longer, more resilient tethers using advanced conductive materials such as carbon nanotubes and graphene composites. These materials promise to enhance current-carrying capacity, reduce mass, and improve resistance to the harsh space environment, addressing one of the primary technical challenges of EDT deployment.
A major research direction involves the integration of EDTs with satellite servicing and debris mitigation missions. By leveraging the ability of tethers to generate thrust or drag through interaction with Earth’s magnetic field, future systems could enable controlled deorbiting of defunct satellites or repositioning of operational assets in geosynchronous orbit (GEO). This capability aligns with the growing emphasis on sustainable space operations and the mitigation of orbital debris, a priority for organizations such as the National Aeronautics and Space Administration (NASA) and the European Space Agency (ESA).
Innovations in autonomous control algorithms and onboard power electronics are also expected to play a pivotal role. Advanced guidance, navigation, and control (GNC) systems will be essential for precise tether deployment, dynamic stability, and real-time adjustment of tether current to optimize thrust or drag. Research initiatives, including those supported by NASA and ESA, are exploring machine learning approaches to enhance the reliability and efficiency of these systems in the complex GEO environment.
Looking further ahead, the long-term impact of geosynchronous orbit EDT systems could be transformative for space infrastructure. The potential for propellantless station-keeping and orbital maneuvering offers a pathway to significantly extend satellite lifespans and reduce operational costs. Moreover, EDTs could facilitate the assembly and maintenance of large-scale platforms in GEO, supporting future communications, Earth observation, and even solar power generation projects. The Japan Aerospace Exploration Agency (JAXA) and other national space agencies are actively investigating these applications as part of broader efforts to enable sustainable and scalable space activities.
- Continued international collaboration and demonstration missions will be critical to validate EDT technologies in operational GEO scenarios.
- Regulatory frameworks and best practices for tether deployment and end-of-life management are expected to evolve in parallel with technical progress.
- As EDT systems mature, their integration into commercial and governmental satellite fleets could become a standard practice, fundamentally reshaping the economics and sustainability of geosynchronous orbit operations.
Sources & References
- NASA
- European Space Agency
- Japan Aerospace Exploration Agency
- NASA
- European Space Agency
- United Nations Office for Outer Space Affairs
- International Telecommunication Union