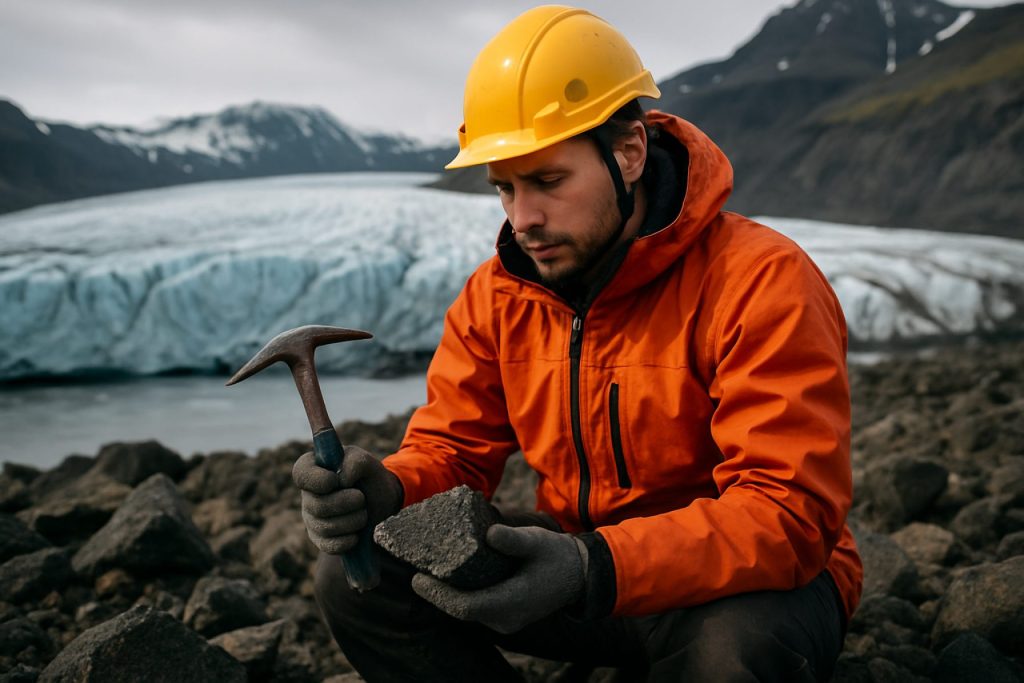
Table of Contents
- Executive Summary: Key Insights for 2025–2030
- Current Landscape: Subglacial Basalt Geochemistry in 2025
- Emerging Technologies: Innovations in Sampling and Analysis
- Market Forecast: Growth Projections and Investment Trends
- Key Players and Research Institutions: Global Leaders Driving Progress
- Industrial Applications: Mining, Resource Exploration, and Climate Implications
- Environmental Impact: Subglacial Basalt Chemistry and Ecosystem Interactions
- Data Integration: AI, Remote Sensing, and Advanced Geochemical Modeling
- Policy, Regulation, and Collaboration: Industry Standards and Global Initiatives
- Future Outlook: Opportunities, Challenges, and the Road Ahead to 2030
- Sources & References
Executive Summary: Key Insights for 2025–2030
Subglacial basalt geochemistry, a field that interrogates the chemical processes and mineral transformations occurring beneath glaciers and ice caps, is poised for significant advancements between 2025 and 2030. The intersection of glaciology, volcanology, and geochemistry is driving new insights into Earth system processes, climate feedbacks, and carbon sequestration potential. The next five years are expected to be marked by enhanced data resolution, expanded sampling campaigns, and a growing focus on the role of subglacial environments in global biogeochemical cycles.
Recent field campaigns beneath the Greenland and Antarctic ice sheets have highlighted the unique geochemical signatures of subglacial basalts, shaped by high-pressure, low-temperature weathering regimes. These settings foster rapid basalt alteration, releasing key ions such as calcium, magnesium, and silica, which are central to natural carbon sequestration processes through silicate weathering. In 2025, research efforts are increasingly leveraging autonomous subglacial sampling technologies and advanced spectroscopic techniques to capture in-situ water-rock interaction data with unprecedented fidelity. These innovations are supported by collaborations among academic institutions and technology providers specializing in polar instrumentation.
A driving force for the increased attention on subglacial basalt geochemistry is its relevance to atmospheric CO2 drawdown, a topic of interest for both scientific and industrial stakeholders. As basalt weathering under glaciers accelerates due to glacial retreat and increased meltwater flux, the resultant fluxes of dissolved cations and secondary mineral formation are being closely monitored. Organizations such as British Antarctic Survey and U.S. Geological Survey are expanding their datasets on subglacial geochemical fluxes, providing a foundation for predictive models assessing the role of subglacial processes in global carbon cycles.
From 2025 onward, the field is expected to benefit from the integration of machine learning with geochemical datasets, enabling more robust predictions of subglacial weathering rates and elemental fluxes under various climate scenarios. Additionally, there is a growing industry interest in leveraging natural basaltic systems for enhanced carbon capture and storage, as evidenced by pilot projects and feasibility studies by groups such as Carbfix, which investigates in-situ mineralization of CO2 in basaltic formations.
In summary, the period from 2025 to 2030 is set to witness a convergence of high-resolution geochemical data, innovative analytical techniques, and applied research into subglacial basalt dynamics. These developments hold promise for both advancing fundamental Earth science and informing scalable climate mitigation strategies.
Current Landscape: Subglacial Basalt Geochemistry in 2025
In 2025, subglacial basalt geochemistry continues to represent a dynamic intersection of volcanology, geochemistry, and glaciology, driven largely by its implications for understanding Earth’s deep carbon cycle, magmatic processes under ice, and the broader context of cryosphere-volcano interactions. Recent advances have been catalyzed by improved in situ sampling technologies and the integration of high-resolution geochemical analysis with remote sensing data.
A key focus in the current landscape is the role of subglacial basalt in carbon sequestration and weathering dynamics. Basaltic rocks beneath glaciers, particularly in Iceland and Antarctica, are now recognized as significant sites for enhanced chemical weathering, which draws down atmospheric CO2 through the formation of carbonate minerals. Field campaigns in regions such as Vatnajökull and Mýrdalsjökull have leveraged autonomous drilling and geochemical sensors to collect pristine basalt samples, revealing elevated rates of silicate weathering and unique trace element mobility attributed to subglacial hydrology and pressure conditions.
Modern analytical platforms, such as inductively coupled plasma mass spectrometry (ICP-MS) and laser ablation systems, are now routinely deployed by leading research consortia and university laboratories, allowing for precise measurements of major and trace elements, as well as isotopic compositions in subglacial basalts. This technological progress has also facilitated the mapping of spatial geochemical variations within subglacial volcanic provinces, enhancing models of magmatic differentiation and crustal assimilation under ice.
International collaborations—often involving agencies like the British Geological Survey and national geological institutes—have intensified, with data sharing agreements and coordinated field studies now standard practice. These efforts are complemented by the emergence of open-access geochemical databases, which are consolidating data from both legacy and newly collected subglacial samples for global comparative analysis.
Looking ahead, the coming years are expected to see a greater integration of geochemical data with physical ice-sheet models, led by projects at institutions such as British Antarctic Survey and U.S. Geological Survey. The goal is to quantify feedbacks between basalt geochemistry, subglacial hydrology, and climate. In parallel, commercial and governmental interest in the potential of subglacial basalt for carbon capture and long-term mineral storage is rising, with pilot projects exploring engineered weathering approaches in glaciated volcanic terrains.
In sum, 2025 marks a phase of rapid methodological innovation and expanding interdisciplinary collaboration in subglacial basalt geochemistry, with both fundamental scientific and applied environmental implications expected to drive research momentum into the next several years.
Emerging Technologies: Innovations in Sampling and Analysis
Emerging technologies are rapidly transforming the field of subglacial basalt geochemistry, particularly in the context of sampling and analytical methods. In 2025, several key advances are enhancing the ability of researchers to access, collect, and characterize subglacial basalt samples with unprecedented precision and efficiency. These developments are propelled by interdisciplinary collaborations between geoscientists, equipment manufacturers, and research consortia.
One notable innovation is the utilization of autonomous and remotely operated drilling systems, designed to withstand the extreme conditions beneath glaciers. Such systems are increasingly being deployed to penetrate thick ice and retrieve core samples of basaltic bedrock. Recent projects in polar regions have demonstrated the feasibility of hot-water drilling combined with robotic coring tools, enabling the extraction of minimally contaminated basalt samples for geochemical analysis. Companies specializing in drilling technology, such as Sandvik, are actively developing equipment tailored for subglacial environments, providing improved drill head materials and onboard sensors for real-time data acquisition.
Advancements in in situ geochemical analysis are also shaping the landscape. Portable X-ray fluorescence (pXRF) and laser-induced breakdown spectroscopy (LIBS) instruments are undergoing significant refinement, allowing researchers to perform preliminary geochemical assessments directly at the drill site. Manufacturers like Thermo Fisher Scientific and Olympus Corporation are at the forefront of producing ruggedized, field-deployable spectrometers that deliver rapid elemental profiling of basalt samples without extensive sample preparation.
Furthermore, the integration of advanced data analytics and remote sensing technologies is improving spatial mapping and characterization of subglacial basaltic terrains. High-resolution satellite imagery and airborne geophysical surveys, supported by organizations such as European Space Agency, are being leveraged to identify promising sampling sites and monitor glacial dynamics that influence basalt exposure and access. These approaches offer a holistic view, enabling researchers to target sampling campaigns more strategically.
Looking ahead, the convergence of robotics, sensor miniaturization, and machine learning is expected to further accelerate progress in subglacial basalt geochemistry. Planned field campaigns through 2026 and beyond are anticipated to deploy next-generation robotic platforms, integrating multi-sensor payloads for autonomous navigation and real-time data relay. These innovations are poised to deepen our understanding of subglacial geochemical processes, with broader implications for volcanology, planetary science, and carbon sequestration research.
Market Forecast: Growth Projections and Investment Trends
The market for subglacial basalt geochemistry is entering a phase of accelerated growth, primarily driven by the increased relevance of carbon sequestration, mineral resource exploration, and climate research. As of 2025, global investment in subglacial basalt studies is expanding, with a focus on understanding the geochemical processes that facilitate both natural and engineered carbon storage in basaltic formations beneath glaciers. The demand is further fueled by the unique ability of subglacial basalts to react with CO₂, converting it into stable carbonate minerals through rapid mineralization—a process being leveraged in pilot projects and commercial ventures worldwide.
Major players in the geosciences and energy sectors are ramping up funding and collaborative research initiatives. For example, several carbon capture and storage (CCS) projects, such as those supported by organizations like Shell and Equinor, are directing attention toward subglacial basalt formations due to their high reactivity and porosity. These companies are investing in field trials and laboratory research to optimize injection and monitoring techniques, aiming to scale up CO₂ sequestration efforts over the next three to five years.
In parallel, government agencies and public research institutions—especially in regions with extensive basalt provinces such as Iceland and parts of North America—are increasing funding for mapping and characterizing subglacial basalts. The U.S. Geological Survey and the Norwegian Geotechnical Institute are among those intensifying geochemical and hydrological studies, with a view toward both environmental monitoring and resource development.
Investment trends show a growing allocation of capital toward advanced analytical technologies, such as isotope geochemistry, in-situ monitoring sensors, and high-resolution subsurface imaging. Instrument manufacturers like Thermo Fisher Scientific are responding with tailored solutions for geochemical analysis, supporting the sector’s expanding needs.
Looking ahead, the subglacial basalt geochemistry market is projected to see double-digit annual growth rates through the late 2020s, propelled by global decarbonization targets and the increasing recognition of mineral carbonation as a viable negative emissions technology. Continued collaboration between industry, academia, and government agencies is expected to accelerate commercialization pathways, while ongoing investment will likely unlock new opportunities for resource assessment, environmental management, and climate solutions.
Key Players and Research Institutions: Global Leaders Driving Progress
Subglacial basalt geochemistry is a highly specialized field that investigates the chemical and isotopic characteristics of basalts formed and altered beneath glaciers and ice sheets. This research is crucial for understanding Earth’s volcanic processes, past climate conditions, and the cycling of elements in extreme environments. As of 2025, several leading research institutions and scientific organizations are at the forefront of advancing knowledge in this area.
In Iceland, the University of Iceland remains a global hub for subglacial basalt research. Its Institute of Earth Sciences continues to spearhead multidisciplinary projects focused on the unique subglacial volcanic systems of Iceland, such as those beneath the Vatnajökull and Mýrdalsjökull ice caps. These efforts include collaborations with international partners to analyze the geochemistry of basalts erupted during subglacial events, using advanced analytical techniques to characterize major, trace, and volatile elements.
In Antarctica, the National Science Foundation (NSF) in the United States funds several initiatives under the United States Antarctic Program, supporting research into subglacial volcanism and its geochemical signatures. Projects like the Subglacial Antarctic Lakes Scientific Access (SALSA) and ongoing work at Mount Erebus are providing key data on the composition and evolution of basalts formed in cryogenic conditions.
The British Antarctic Survey (BAS) is another prominent player, leading investigations into the geochemistry of subglacial volcanic rocks in West Antarctica. BAS’s work emphasizes the links between volcanic activity, ice sheet stability, and geochemical fluxes to the Southern Ocean, with implications for understanding global biogeochemical cycles.
In North America, the Natural Resources Canada (NRCan) and several Canadian universities are leveraging Canada’s extensive glaciated volcanic terrains, such as the Mount Edziza and Wells Gray–Clearwater volcanic fields, to investigate interactions between ice and basaltic magmatism. These studies increasingly utilize drone-based sampling and high-resolution geochemical mapping.
Looking ahead, the next few years will see intensified international collaboration, especially in the context of climate change and carbon sequestration research. Organizations like Orkuveita Reykjavíkur in Iceland are exploring the role of subglacial basalts in natural carbon capture and storage, building on their experience with the CarbFix project. This intersection of geochemistry, volcanology, and environmental science is expected to drive new research directions and technology development through 2025 and beyond.
Industrial Applications: Mining, Resource Exploration, and Climate Implications
Subglacial basalt geochemistry is gaining prominence in industrial sectors such as mining, resource exploration, and climate mitigation, owing to its unique mineralogical and geochemical signatures. In 2025, the focus on subglacial basalts is intensifying, particularly due to their potential as repositories for critical metals, their role as analogs for hidden ore deposits, and their interaction with carbon sequestration processes.
Subglacial basalt formations, especially those in regions like Iceland and Antarctica, are of increasing interest for mining and exploration firms seeking strategic resources such as nickel, copper, cobalt, and platinum-group elements. These metals are essential for battery technologies and the energy transition. Basalts formed under glacial ice exhibit distinct geochemical markers—such as elevated concentrations of incompatible elements and unique isotopic ratios—that can help geologists trace hydrothermal alteration zones and locate concealed ore systems. Exploration initiatives in 2025 are leveraging advanced geochemical mapping and remote sensing technologies to identify subglacial volcanic terrains with the highest resource potential. Companies specializing in green energy minerals are collaborating with geological surveys to assess new targets and refine extraction techniques.
A particularly significant development is the deployment of subglacial basalt reservoirs for industrial-scale carbon sequestration. Basalt’s high reactivity with CO2 enables accelerated mineral carbonation—a process where CO2 is permanently locked into stable carbonate minerals. In 2025, pilot projects are expanding, with companies such as Carbfix and Climeworks advancing partnerships to inject captured atmospheric CO2 into subglacial basalt formations. These efforts are supported by ongoing research collaborations with government and academic institutions, targeting both the scalability and long-term monitoring of carbon mineralization in cold, ice-proximal settings.
From a resource exploration perspective, subglacial basalt geochemistry is also informing the development of new geophysical and geochemical survey protocols. As exploration moves into increasingly remote and environmentally sensitive regions, non-invasive techniques—such as drone-based hyperspectral imaging and portable X-ray fluorescence (pXRF)—are being adapted to subglacial terrains. The data gathered from these studies not only guides industrial exploration but also enhances understanding of glacial-volcanic interactions, which can inform hazard assessment and infrastructure planning in polar regions.
Looking ahead, the integration of subglacial basalt geochemistry into industrial applications is expected to expand, driven by the dual imperatives of resource security and climate action. As companies and governments seek to balance extraction with environmental stewardship, the geochemical study of subglacial basalts will remain central to both sustainable mining strategies and the development of effective carbon sequestration technologies.
Environmental Impact: Subglacial Basalt Chemistry and Ecosystem Interactions
Subglacial basalt geochemistry is increasingly recognized as a key driver of environmental processes in glacial and subglacial ecosystems, with new research in 2025 focusing on its influence on biogeochemical cycling and downstream ecosystem health. When glaciers override basaltic bedrock, interactions between meltwater and basalt produce a suite of dissolved ions, nutrients, and trace metals. These geochemical transformations are critical in cold regions such as Iceland, Antarctica, and Greenland, where extensive basaltic provinces exist beneath ice cover.
Recent field campaigns and laboratory studies have demonstrated that subglacial weathering of basalt releases significant quantities of elements such as iron, magnesium, silica, and phosphorus into meltwaters. These nutrients can support microbial productivity both beneath the ice and in proglacial rivers, influencing local food webs and carbon cycling. In particular, iron released from basalt weathering is now shown to be bioavailable, supporting primary productivity as meltwater enters downstream marine environments—a factor of growing interest in the context of climate-driven glacial melt acceleration (British Antarctic Survey).
The environmental impact of subglacial basalt geochemistry extends to the regulation of atmospheric CO₂ via enhanced silicate weathering. As meltwater interacts with basalt, carbon dioxide is consumed through chemical reactions, contributing to long-term carbon sequestration. Field data collected in 2024 and early 2025 highlight that this process is highly dynamic, with rates increasing alongside glacier retreat and higher meltwater fluxes (U.S. Geological Survey). This has spurred interest in the potential for engineered enhancements of basalt weathering as a negative emissions technology, with several pilot projects planned in basalt-rich glacial catchments over the next few years.
However, there remain important uncertainties regarding the ecological impacts of changing subglacial geochemical fluxes. Rapidly increasing nutrient and metal loads could alter microbial community composition and biogeochemical cycling, with downstream consequences for both freshwater and coastal ecosystems. Monitoring programs initiated by governmental and scientific agencies are expanding, using sensors and autonomous platforms to track chemical outputs from subglacial environments in real time (NASA).
Looking ahead, interdisciplinary collaborations are set to intensify, combining geochemistry, microbiology, and remote sensing to resolve the feedbacks between subglacial basalt weathering, ecosystem function, and climate. The next few years will likely see advances in predictive modeling, improved quantification of nutrient and trace metal fluxes, and a better understanding of the role of subglacial environments in Earth’s changing carbon and nutrient cycles.
Data Integration: AI, Remote Sensing, and Advanced Geochemical Modeling
The integration of artificial intelligence (AI), remote sensing technologies, and advanced geochemical modeling is rapidly transforming the study of subglacial basalt geochemistry. As of 2025, interdisciplinary efforts are leveraging these tools to address the extreme logistical and analytical challenges inherent in accessing and interpreting geochemical data from subglacial volcanic environments, such as those beneath Icelandic ice caps or the Antarctic ice sheet.
Remote sensing platforms, including satellite-based hyperspectral imaging and airborne radar, are now providing unprecedented spatial and temporal resolution of subglacial volcanic systems. These technologies enable the indirect assessment of basaltic rock composition and alteration patterns, even beneath thick ice cover. Leading organizations such as European Space Agency and NASA continue to develop and deploy advanced sensors capable of mapping geothermal anomalies, meltwater pathways, and mineralogical variations associated with subglacial basaltic terrains.
AI-driven data analytics are pivotal in synthesizing these massive remote sensing datasets with direct geochemical measurements collected from ice cores, boreholes, and subglacial outflows. Machine learning algorithms are being trained to identify key geochemical signatures—such as trace element ratios, isotopic compositions, and alteration indices—indicative of basalt-water interactions and hydrothermal alteration beneath glaciers. These approaches accelerate pattern recognition and anomaly detection, guiding targeted sampling campaigns and improving model accuracy.
Advanced geochemical modeling platforms, often cloud-based and equipped with AI-enhanced simulation capabilities, are now integrating multi-source datasets to simulate subglacial basaltic processes. These models incorporate real-time environmental data, such as temperature, pressure, and fluid chemistry, to predict mineralogical transformations, element mobility, and the generation of secondary minerals under dynamic subglacial conditions. Initiatives by research consortia, in collaboration with technology providers like IBM and Microsoft, are driving the deployment of scalable geochemical modeling environments tailored to glaciovolcanic systems.
Looking ahead, the next few years are expected to witness further advances in autonomous sensor deployment—such as instrumented subglacial drones and borehole robots—capable of collecting high-resolution, in situ geochemical data from previously inaccessible environments. These will feed directly into AI-driven analytical pipelines, offering real-time, adaptive modeling of subglacial basalt geochemistry and its implications for global biogeochemical cycles, mineral resource assessment, and volcanic hazard forecasting.
Policy, Regulation, and Collaboration: Industry Standards and Global Initiatives
The regulatory and policy landscape surrounding subglacial basalt geochemistry is rapidly evolving as interest in the environmental, industrial, and climate-related applications of basaltic materials intensifies. In 2025, international collaboration, standardization efforts, and new regulatory frameworks are being shaped by the growing recognition of subglacial basalt’s potential in carbon sequestration, mineral extraction, and geoengineering initiatives.
One of the most significant developments is the increasing alignment of geochemical research with global climate targets. Organizations such as the Intergovernmental Panel on Climate Change (IPCC) have highlighted the role of enhanced weathering and mineral carbonation, particularly using basalt, as a negative emissions technology. This has prompted governments and industry to explore regulatory pathways for safe and effective deployment, especially in sensitive subglacial environments where unique geochemical processes prevail.
Industry standards are under active development, with bodies like the International Organization for Standardization (ISO) and CSA Group engaging in technical committee work to define sampling protocols, analytical methods, and reporting requirements specific to basaltic geochemistry. These standards aim to ensure the reproducibility and comparability of subglacial basalt data, crucial for both scientific integrity and regulatory compliance.
On the policy front, several Arctic and sub-Arctic nations are updating their environmental impact assessment (EIA) frameworks to address mineral extraction and carbon sequestration projects targeting subglacial basalt formations. These updates reflect concerns about potential impacts on glacial hydrology, local ecosystems, and indigenous communities. For instance, the Natural Resources Canada and counterparts in Iceland and Greenland are working on cross-border guidelines that account for the unique geochemical and environmental dynamics of subglacial basalt regions.
Collaboration is also evident in multi-stakeholder initiatives. Research consortia involving universities, government agencies, and industry partners are coordinating field studies in Greenland and Antarctica, sharing geochemical datasets, and harmonizing methodologies. The British Antarctic Survey and U.S. Geological Survey (USGS) are among the organizations leading these collaborative efforts, which are expected to set precedents for global best practices in subglacial basalt research and resource management over the next few years.
Looking ahead, the outlook for policy and regulation in subglacial basalt geochemistry is one of increasing stringency, transparency, and international cooperation. As scientific understanding deepens and industrial applications expand, proactive standard-setting and collaborative governance will be essential to balancing innovation with environmental stewardship.
Future Outlook: Opportunities, Challenges, and the Road Ahead to 2030
The future of subglacial basalt geochemistry is poised at a dynamic intersection of technological innovation and growing global interest in climate-resilient geosciences. As of 2025, research efforts are intensifying to unravel the unique interactions between basaltic bedrock and glacial environments, driven by both fundamental scientific inquiry and practical applications in carbon sequestration, resource exploration, and paleoclimate reconstruction.
One major opportunity lies in leveraging subglacial basalt as a natural medium for carbon dioxide mineralization. Studies have shown that the rapid weathering of basalt beneath glaciers can enhance the uptake of atmospheric CO2 through the formation of stable carbonate minerals. This process, known as enhanced rock weathering, is being explored by organizations such as CarbonCure Technologies and Climeworks, which are developing scalable carbon capture solutions based on mineralization, although their current commercial focus is not explicitly subglacial. The coming years are expected to see pilot projects aiming to quantify the efficacy and scalability of subglacial basalt weathering as a viable carbon sink.
Technological advancements in geochemical sampling and remote sensing are rapidly expanding our ability to access and analyze subglacial environments. The deployment of autonomous subglacial drilling systems and in-situ geochemical sensors is anticipated to generate high-resolution datasets on water-rock interactions, trace element fluxes, and isotopic signatures. Companies such as Kongsberg Gruppen and Sandvik are at the forefront of developing drilling and sensor technologies for extreme environments, which could be adapted for cryospheric applications.
However, significant challenges remain. The logistical complexity and high cost of subglacial fieldwork limit the frequency and scope of direct sampling campaigns. Environmental stewardship is also a concern, as increased scientific activity must be balanced with the preservation of pristine polar ecosystems. Regulatory oversight from entities like the British Antarctic Survey and National Science Foundation is expected to guide responsible research protocols.
Looking toward 2030, the road ahead for subglacial basalt geochemistry will likely be shaped by sustained interdisciplinary collaboration, integration of real-time data analytics, and the development of robust models to predict geochemical fluxes under changing climatic conditions. As the field advances, it will not only enhance our understanding of Earth’s biogeochemical cycles but also inform strategies for climate mitigation and sustainable resource management.
Sources & References
- British Antarctic Survey
- Carbfix
- British Geological Survey
- Sandvik
- Thermo Fisher Scientific
- Olympus Corporation
- European Space Agency
- Shell
- Equinor
- University of Iceland
- National Science Foundation
- Natural Resources Canada
- Orkuveita Reykjavíkur
- Climeworks
- NASA
- IBM
- Microsoft
- Intergovernmental Panel on Climate Change
- International Organization for Standardization
- CSA Group
- Kongsberg Gruppen