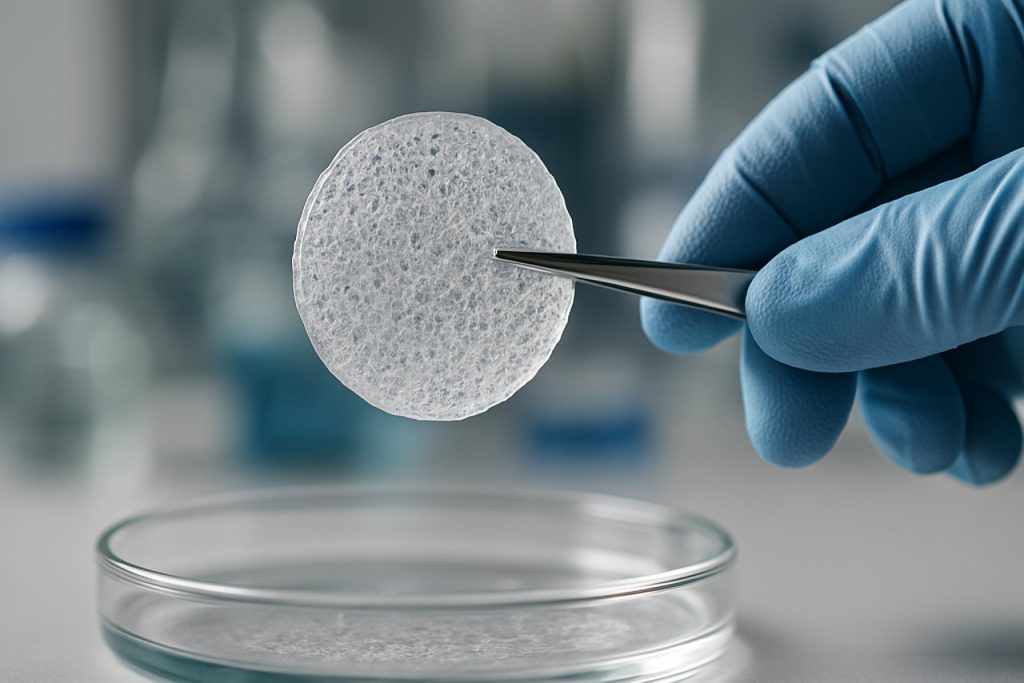
Polysaccharide-Based Hydrogel Scaffolds for Tissue Engineering in 2025: Unleashing Next-Gen Regenerative Medicine and Market Growth. Explore How Advanced Biomaterials Are Shaping the Future of Healthcare Innovation.
- Executive Summary and 2025 Market Outlook
- Key Drivers: Medical Demand and Biocompatibility Advantages
- Market Size, Segmentation, and 2025–2030 Growth Forecasts
- Technological Innovations in Polysaccharide Hydrogel Scaffold Design
- Leading Companies and Strategic Partnerships (e.g., regenemed.com, gelita.com)
- Regulatory Landscape and Quality Standards (e.g., fda.gov, iso.org)
- Emerging Applications: From Orthopedics to Organ Regeneration
- Challenges: Manufacturing, Scalability, and Clinical Translation
- Regional Analysis: North America, Europe, Asia-Pacific Trends
- Future Outlook: Disruptive Technologies and Projected CAGR (2025–2030)
- Sources & References
Executive Summary and 2025 Market Outlook
Polysaccharide-based hydrogel scaffolds have emerged as a pivotal class of biomaterials in the field of tissue engineering, offering biocompatibility, tunable mechanical properties, and the ability to mimic the extracellular matrix. As of 2025, the sector is experiencing accelerated growth, driven by advances in regenerative medicine, increased investment in biomaterials R&D, and a rising demand for personalized healthcare solutions. Key polysaccharides utilized include alginate, chitosan, hyaluronic acid, and cellulose derivatives, each contributing unique properties to scaffold design and function.
Major industry players are expanding their portfolios to address the growing need for advanced scaffolds. Thermo Fisher Scientific continues to supply a broad range of research-grade polysaccharides and hydrogel kits, supporting both academic and industrial tissue engineering projects. Merck KGaA (operating as Sigma-Aldrich) remains a leading supplier of high-purity biopolymers and custom hydrogel formulations, catering to the evolving requirements of scaffold fabrication and cell culture. Cytiva (formerly GE Healthcare Life Sciences) is also notable for its biomaterials and cell culture solutions, which are increasingly being integrated into scaffold development pipelines.
Recent years have seen a surge in collaborative efforts between biomaterials companies and medical device manufacturers to accelerate clinical translation. For example, Evonik Industries has expanded its biomaterials division, focusing on medical-grade polysaccharides and custom hydrogel systems for tissue regeneration applications. Meanwhile, DuPont is leveraging its expertise in cellulose and hyaluronic acid derivatives to develop next-generation scaffolds with enhanced bioactivity and mechanical strength.
The regulatory landscape is also evolving, with agencies such as the U.S. Food and Drug Administration (FDA) and the European Medicines Agency (EMA) providing clearer pathways for the approval of advanced biomaterials and combination products. This is expected to facilitate faster market entry for innovative polysaccharide-based scaffolds, particularly those designed for wound healing, cartilage repair, and soft tissue regeneration.
Looking ahead to the next few years, the market outlook remains robust. The integration of 3D bioprinting and advanced manufacturing techniques is anticipated to further drive adoption, enabling the production of patient-specific scaffolds with precise architecture and functionality. Strategic partnerships, increased funding, and ongoing technological innovation position polysaccharide-based hydrogel scaffolds as a cornerstone of the tissue engineering sector through 2025 and beyond.
Key Drivers: Medical Demand and Biocompatibility Advantages
The demand for advanced tissue engineering solutions is intensifying in 2025, driven by a global rise in chronic diseases, trauma cases, and the need for organ and tissue regeneration. Polysaccharide-based hydrogel scaffolds are at the forefront of this trend, primarily due to their exceptional biocompatibility, tunable physical properties, and ability to mimic the natural extracellular matrix (ECM). These materials, derived from natural sources such as alginate, chitosan, hyaluronic acid, and cellulose, are increasingly favored in clinical and research settings for their low immunogenicity and capacity to support cell adhesion, proliferation, and differentiation.
A key driver is the growing prevalence of conditions requiring regenerative therapies, such as osteoarthritis, cardiovascular diseases, and diabetic wounds. The World Health Organization projects a continued increase in non-communicable diseases globally, further fueling the need for innovative biomaterials. Polysaccharide-based hydrogels are uniquely positioned to address these needs, as they can be engineered to deliver bioactive molecules, support vascularization, and degrade in a controlled manner, aligning with the healing process.
Biocompatibility remains a central advantage. Unlike many synthetic polymers, polysaccharide hydrogels are less likely to provoke adverse immune responses, making them suitable for a wide range of tissue engineering applications. Companies such as Lonza Group and Cytiva (formerly GE Healthcare Life Sciences) are actively developing and supplying high-purity polysaccharide materials for research and clinical use. Sigma-Aldrich (now part of Merck KGaA) also provides a broad portfolio of polysaccharide-based hydrogel products, supporting both academic and industrial R&D.
In 2025, the scalability and reproducibility of these hydrogels are being enhanced through advances in manufacturing technologies, including 3D bioprinting and automated hydrogel synthesis. Companies like CELLINK are pioneering bioprinting platforms that utilize polysaccharide-based bioinks, enabling the fabrication of complex, patient-specific scaffolds for tissue repair and organoid development.
Looking ahead, the next few years are expected to see further integration of polysaccharide-based hydrogels with smart biomaterials and bioactive agents, expanding their therapeutic potential. Regulatory support for biocompatible, naturally derived scaffolds is also anticipated to strengthen, as safety and efficacy data accumulate. Collectively, these drivers position polysaccharide-based hydrogel scaffolds as a cornerstone technology in the evolving landscape of tissue engineering and regenerative medicine.
Market Size, Segmentation, and 2025–2030 Growth Forecasts
The global market for polysaccharide-based hydrogel scaffolds in tissue engineering is poised for robust growth from 2025 through 2030, driven by increasing demand for regenerative medicine solutions, advancements in biomaterials, and expanding clinical applications. As of 2025, the market is characterized by a diverse segmentation based on source polysaccharide (such as alginate, chitosan, hyaluronic acid, cellulose, and dextran), application (bone, cartilage, wound healing, dental, and soft tissue engineering), and end-user (hospitals, research institutes, and specialty clinics).
Alginate and chitosan hydrogels remain the most widely adopted, owing to their biocompatibility, tunable mechanical properties, and ease of functionalization. Companies such as NovaMatrix (a business unit of DSM), a leading supplier of ultrapure alginates and chitosan, continue to expand their product portfolios to meet the evolving needs of tissue engineering researchers and clinicians. Sigma-Aldrich (now part of Merck KGaA) and GELITA also play significant roles in supplying high-purity polysaccharides and hydrogel precursors for scaffold fabrication.
Regionally, North America and Europe are expected to maintain dominance due to strong R&D infrastructure, supportive regulatory frameworks, and the presence of major biomaterials manufacturers. However, Asia-Pacific is projected to witness the fastest growth, fueled by increasing investments in healthcare infrastructure, rising adoption of advanced wound care, and government initiatives supporting regenerative medicine.
From 2025 to 2030, the market is forecasted to grow at a compound annual growth rate (CAGR) in the high single digits, with estimates from industry sources and company reports suggesting the global market value could surpass USD 1.5 billion by 2030. This growth is underpinned by the expanding pipeline of clinical trials utilizing polysaccharide-based scaffolds for applications such as cartilage repair, chronic wound management, and dental tissue regeneration. Companies like Advanced BioMatrix and CELLINK (a BICO company) are actively developing and commercializing next-generation hydrogel scaffolds tailored for 3D bioprinting and patient-specific tissue constructs.
Looking ahead, the market outlook remains positive, with anticipated breakthroughs in scaffold customization, bioactive molecule incorporation, and scalable manufacturing. Strategic collaborations between material suppliers, device manufacturers, and clinical research organizations are expected to accelerate the translation of polysaccharide-based hydrogel scaffolds from bench to bedside, further expanding their role in the future of tissue engineering.
Technological Innovations in Polysaccharide Hydrogel Scaffold Design
The landscape of polysaccharide-based hydrogel scaffold design for tissue engineering is experiencing rapid technological innovation as of 2025, driven by advances in material science, biofabrication, and clinical translation. Polysaccharides such as alginate, chitosan, hyaluronic acid, and cellulose derivatives are at the forefront due to their biocompatibility, tunable properties, and abundance. Recent years have seen a surge in the development of smart hydrogels with enhanced mechanical strength, bioactivity, and responsiveness to physiological stimuli.
A key trend is the integration of advanced crosslinking techniques, including enzymatic, photo-crosslinking, and click chemistry approaches, which allow for precise control over scaffold architecture and degradation rates. For example, companies like Lonza Group and Sigma-Aldrich (now part of Merck KGaA) supply high-purity polysaccharides and crosslinkers tailored for biomedical hydrogel applications, supporting both research and clinical-grade production.
3D bioprinting is another transformative innovation, enabling the fabrication of patient-specific scaffolds with complex geometries and spatially controlled bioactive cues. Leading bioprinting technology providers such as CELLINK (a BICO company) and Organovo Holdings are actively developing bioinks based on polysaccharide hydrogels, facilitating the creation of functional tissue constructs for regenerative medicine and drug testing. These bioinks are engineered for optimal printability, cell viability, and post-printing stability, addressing longstanding challenges in scaffold reproducibility and scalability.
In parallel, the incorporation of nanomaterials and bioactive molecules into polysaccharide hydrogels is enhancing their functionality. For instance, the addition of nanocellulose or graphene oxide can significantly improve mechanical properties and promote cell adhesion, while the controlled release of growth factors or peptides supports tissue regeneration. Companies such as GELITA and Ashland Global Holdings are expanding their portfolios to include functionalized polysaccharide derivatives and composite hydrogels for advanced biomedical applications.
Looking ahead, the next few years are expected to bring further convergence of hydrogel scaffold design with digital health and personalized medicine. The integration of biosensors and real-time monitoring capabilities into polysaccharide hydrogels is under active exploration, aiming to provide dynamic feedback on tissue regeneration and scaffold performance. As regulatory pathways for advanced biomaterials become clearer, industry leaders and startups alike are poised to accelerate the clinical translation of polysaccharide-based hydrogel scaffolds, with a focus on musculoskeletal, neural, and soft tissue engineering.
Leading Companies and Strategic Partnerships (e.g., regenemed.com, gelita.com)
The landscape of polysaccharide-based hydrogel scaffolds for tissue engineering in 2025 is shaped by a dynamic interplay of established biomaterials manufacturers, innovative startups, and strategic collaborations with research institutions. These partnerships are accelerating the translation of hydrogel technologies from laboratory research to clinical and commercial applications, particularly in regenerative medicine, wound healing, and 3D bioprinting.
Among the leading companies, GELITA AG stands out as a global supplier of collagen and gelatin biomaterials, with a growing portfolio that includes polysaccharide-based hydrogels. GELITA’s expertise in biopolymer processing and its collaborations with medical device manufacturers have enabled the development of advanced scaffolds tailored for soft tissue regeneration and wound care. The company’s ongoing investments in R&D and partnerships with academic centers are expected to yield new hydrogel formulations with enhanced biocompatibility and tunable mechanical properties over the next few years.
Another notable player is Regenemed, which specializes in tissue engineering scaffolds and regenerative medicine solutions. Regenemed’s focus on polysaccharide-based hydrogels, such as those derived from alginate and chitosan, has led to the commercialization of customizable scaffold platforms for cell culture and tissue repair. The company’s strategic alliances with biotechnology firms and clinical research organizations are facilitating the integration of hydrogel scaffolds into next-generation therapies, including stem cell delivery and organoid development.
In the North American market, Lifecore Biomedical is recognized for its production of high-purity hyaluronic acid, a key polysaccharide used in hydrogel scaffold fabrication. Lifecore’s partnerships with medical device companies and pharmaceutical firms are driving the adoption of hyaluronic acid-based hydrogels in both research and clinical settings, particularly for cartilage repair and ophthalmic applications.
Strategic partnerships are also evident in the activities of Evonik Industries, a major specialty chemicals company with a dedicated healthcare division. Evonik’s collaborations with startups and research institutes are focused on developing multifunctional hydrogel scaffolds that combine polysaccharides with synthetic polymers, aiming to optimize cell adhesion, degradation rates, and drug delivery capabilities.
Looking ahead, the next few years are expected to see intensified collaboration between material suppliers, device manufacturers, and clinical partners. These alliances will likely accelerate regulatory approvals and the commercialization of polysaccharide-based hydrogel scaffolds, expanding their use in personalized medicine and complex tissue engineering applications.
Regulatory Landscape and Quality Standards (e.g., fda.gov, iso.org)
The regulatory landscape for polysaccharide-based hydrogel scaffolds in tissue engineering is evolving rapidly as these biomaterials transition from research laboratories to clinical and commercial applications. In 2025, regulatory agencies such as the U.S. Food and Drug Administration (FDA) and international standards organizations like the International Organization for Standardization (ISO) are playing pivotal roles in shaping the requirements for safety, efficacy, and quality.
Polysaccharide-based hydrogels, including those derived from alginate, chitosan, hyaluronic acid, and cellulose, are typically classified as medical devices or combination products, depending on their intended use and incorporation of cells or bioactive agents. In the United States, the FDA’s Center for Devices and Radiological Health (CDRH) oversees the premarket review of such scaffolds. The FDA’s 510(k) premarket notification pathway remains the most common route for devices that demonstrate substantial equivalence to a legally marketed predicate, while the more rigorous Premarket Approval (PMA) process is required for novel or high-risk applications. In 2024 and 2025, the FDA has emphasized the importance of biocompatibility testing (per ISO 10993), sterility assurance, and mechanical performance data for hydrogel scaffolds, as well as clear evidence of their degradation profiles and potential immunogenicity.
Globally, ISO standards are increasingly referenced in regulatory submissions and quality management systems. ISO 13485:2016 remains the benchmark for medical device quality management, while ISO 10993 series addresses biological evaluation of medical devices, including cytotoxicity, sensitization, and genotoxicity testing. For tissue-engineered products, ISO/TS 20399 provides guidance on the characterization of biomaterials and scaffolds, which is particularly relevant for polysaccharide-based hydrogels. The harmonization of these standards across regions is expected to continue, facilitating international market access and reducing duplicative testing.
In the European Union, the Medical Device Regulation (MDR 2017/745) has introduced stricter requirements for clinical evidence and post-market surveillance, impacting manufacturers of hydrogel scaffolds. Companies must now provide robust clinical data and implement comprehensive risk management processes. Notably, several leading biomaterials manufacturers, such as Cytiva (formerly part of GE Healthcare Life Sciences), Lonza, and Evonik Industries, are actively aligning their product development and quality systems with these evolving standards to ensure compliance and facilitate regulatory approvals.
Looking ahead, regulatory agencies are expected to issue further guidance specific to advanced biomaterials and combination products, reflecting the growing complexity of tissue engineering solutions. Stakeholders anticipate increased collaboration between regulators, industry, and standards bodies to address emerging challenges such as personalized scaffolds, 3D bioprinting, and integration of living cells. As the field matures, adherence to rigorous regulatory and quality standards will remain a critical determinant of successful clinical translation and commercialization of polysaccharide-based hydrogel scaffolds.
Emerging Applications: From Orthopedics to Organ Regeneration
Polysaccharide-based hydrogel scaffolds are rapidly advancing as a cornerstone technology in tissue engineering, with 2025 marking a pivotal year for their translation from laboratory research to clinical and commercial applications. These hydrogels, derived from natural polymers such as alginate, chitosan, hyaluronic acid, and cellulose, offer biocompatibility, tunable mechanical properties, and the ability to mimic the extracellular matrix—making them highly attractive for regenerative medicine.
In orthopedics, polysaccharide-based hydrogels are being engineered to support bone and cartilage regeneration. Companies like Gelita AG and DuPont are actively developing and supplying medical-grade polysaccharides and hydrogel formulations for use in bone void fillers and cartilage repair matrices. These scaffolds can be loaded with growth factors or stem cells, enhancing osteogenic and chondrogenic differentiation. Recent preclinical studies have demonstrated that injectable chitosan and alginate hydrogels can promote bone healing and integration with host tissue, with several products entering early-stage clinical trials in Europe and Asia.
Beyond orthopedics, the versatility of polysaccharide hydrogels is driving innovation in soft tissue engineering and organ regeneration. For example, Evonik Industries is leveraging its expertise in biomaterials to develop hyaluronic acid-based hydrogels for wound healing and soft tissue reconstruction. These materials are being tailored for controlled degradation and cell-specific bioactivity, supporting applications in skin, nerve, and vascular tissue engineering. In parallel, Lonza Group is supplying pharmaceutical-grade alginates and other polysaccharides for use in 3D bioprinting, enabling the fabrication of complex, cell-laden constructs for organoid and tissue model development.
A particularly promising frontier is the use of polysaccharide hydrogels as bioinks for 3D bioprinting of functional tissues and organoids. Companies such as CELLINK (a BICO company) are commercializing advanced hydrogel bioinks based on alginate, hyaluronic acid, and cellulose derivatives, which are compatible with a range of cell types and printing modalities. These bioinks are being adopted by research institutions and biotech firms worldwide to create vascularized tissue constructs, liver and kidney organoids, and even early-stage heart tissue models.
Looking ahead to the next few years, the outlook for polysaccharide-based hydrogel scaffolds is robust. Regulatory approvals for hydrogel-based implants and wound care products are expected to increase, particularly in the US, EU, and Asia-Pacific regions. Ongoing collaborations between material suppliers, device manufacturers, and clinical partners are accelerating the pathway from bench to bedside. As manufacturing processes become more standardized and scalable, and as clinical data accumulates, polysaccharide hydrogels are poised to play a transformative role in personalized medicine, organ regeneration, and the broader field of tissue engineering.
Challenges: Manufacturing, Scalability, and Clinical Translation
The translation of polysaccharide-based hydrogel scaffolds from laboratory research to clinical and commercial applications in tissue engineering faces several significant challenges, particularly in the areas of manufacturing, scalability, and regulatory approval. As of 2025, the field is witnessing increased investment and collaboration between academic groups and industry, but key hurdles remain.
One of the primary challenges is the reproducible large-scale manufacturing of hydrogels with consistent physicochemical properties. Polysaccharides such as alginate, chitosan, hyaluronic acid, and cellulose derivatives are inherently variable due to their natural origins, leading to batch-to-batch inconsistencies. Companies like Sigma-Aldrich (now part of Merck KGaA) and Carbosynth supply research-grade polysaccharides, but the transition to medical-grade, GMP-compliant materials suitable for human use requires rigorous purification and quality control processes. This is particularly critical for scaffolds intended for implantation, where even trace contaminants can provoke immune responses or compromise biocompatibility.
Scalability is another pressing issue. While 3D bioprinting and advanced crosslinking techniques have enabled the fabrication of complex hydrogel architectures at the laboratory scale, translating these methods to industrial-scale production remains challenging. Companies such as CELLINK (a BICO company) are developing bioprinting platforms and bioinks specifically tailored for tissue engineering, including polysaccharide-based formulations. However, ensuring uniformity in scaffold structure, mechanical properties, and cell distribution at larger volumes is still a technical bottleneck. Automation and in-line quality monitoring are being integrated into manufacturing workflows, but widespread adoption is expected to take several more years.
Clinical translation is further complicated by regulatory requirements. Polysaccharide-based hydrogels must demonstrate not only safety and efficacy but also long-term stability and predictable degradation profiles. Regulatory agencies such as the U.S. Food and Drug Administration (FDA) and the European Medicines Agency (EMA) require comprehensive preclinical and clinical data, which can be time-consuming and costly to generate. Some companies, including GELITA and Lifecore Biomedical, are actively engaged in developing and supplying medical-grade hydrogels and have experience navigating regulatory pathways, but the approval process for novel tissue engineering products remains lengthy.
Looking ahead, the next few years are likely to see advances in raw material standardization, process automation, and regulatory harmonization. Industry consortia and public-private partnerships are expected to play a key role in addressing these challenges, accelerating the path from bench to bedside for polysaccharide-based hydrogel scaffolds in tissue engineering.
Regional Analysis: North America, Europe, Asia-Pacific Trends
The landscape for polysaccharide-based hydrogel scaffolds in tissue engineering is evolving rapidly across North America, Europe, and Asia-Pacific, with each region demonstrating unique trends and growth drivers as of 2025 and looking ahead.
North America remains a global leader in the development and commercialization of advanced biomaterials, including polysaccharide-based hydrogels. The United States, in particular, benefits from a robust ecosystem of biotechnology firms, academic research centers, and regulatory support. Companies such as DuPont and DSM (now part of dsm-firmenich) are actively involved in the development and supply of biopolymer materials suitable for medical applications. The region’s focus on regenerative medicine, coupled with significant funding from both public and private sectors, is accelerating the translation of hydrogel scaffold technologies from laboratory to clinical settings. The presence of leading medical device manufacturers and a strong intellectual property framework further support innovation and commercialization.
Europe is characterized by a strong emphasis on sustainability and biocompatibility in biomaterials research. The European Union’s regulatory environment, particularly the Medical Device Regulation (MDR), is shaping the development and approval pathways for new hydrogel-based scaffolds. Companies such as Evonik Industries and BASF are notable for their investments in biopolymer research, including polysaccharide derivatives for medical use. Collaborative projects between universities, research institutes, and industry are common, with a focus on applications such as wound healing, cartilage repair, and drug delivery. The region is also witnessing increased activity in start-ups and SMEs leveraging local funding initiatives and EU research grants to advance hydrogel scaffold technologies.
Asia-Pacific is emerging as a dynamic market, driven by expanding healthcare infrastructure, rising investments in biotechnology, and a growing focus on regenerative medicine. Countries like China, Japan, and South Korea are investing heavily in biomaterials research, with government-backed programs supporting innovation. Companies such as Shandong Haohua Chemical Group in China and Nippon Kayaku in Japan are involved in the production and supply of polysaccharide-based materials, including those suitable for medical applications. The region is also seeing increased collaboration between academia and industry, with a focus on cost-effective manufacturing and scaling up production to meet both domestic and international demand.
Looking forward, all three regions are expected to experience continued growth in the adoption of polysaccharide-based hydrogel scaffolds, driven by advances in material science, supportive regulatory frameworks, and increasing demand for tissue engineering solutions. Strategic partnerships, cross-border collaborations, and ongoing investment in R&D will be critical in shaping the competitive landscape through 2025 and beyond.
Future Outlook: Disruptive Technologies and Projected CAGR (2025–2030)
The future outlook for polysaccharide-based hydrogel scaffolds in tissue engineering is marked by rapid technological advancements and a strong projected growth trajectory through 2030. As of 2025, the sector is witnessing disruptive innovations in scaffold design, functionalization, and manufacturing, driven by the convergence of biomaterials science, 3D bioprinting, and regenerative medicine. Polysaccharide hydrogels—derived from sources such as alginate, chitosan, hyaluronic acid, and cellulose—are increasingly favored for their biocompatibility, tunable mechanical properties, and ability to mimic the extracellular matrix.
A key disruptive technology shaping the field is the integration of advanced 3D bioprinting with polysaccharide-based hydrogels. Companies like CELLINK (now part of BICO Group), a global leader in bioprinting, are actively developing bioinks based on natural polysaccharides, enabling the fabrication of complex, patient-specific tissue constructs. These bioinks are engineered to support cell viability and differentiation, crucial for applications in skin, cartilage, and even organoid development. Similarly, Thermo Fisher Scientific is expanding its portfolio of hydrogel materials and cell culture solutions, supporting both research and clinical translation.
Another area of innovation is the functionalization of polysaccharide hydrogels with bioactive molecules, growth factors, and nanoparticles to enhance cell signaling and tissue integration. Companies such as Sigma-Aldrich (a subsidiary of Merck KGaA) supply a wide range of functionalized polysaccharides and hydrogel kits, facilitating rapid prototyping and customization for specific tissue engineering needs. The development of “smart” hydrogels—responsive to stimuli such as pH, temperature, or enzymatic activity—is also gaining momentum, with research collaborations between industry and academic institutions accelerating commercialization.
From a market perspective, the polysaccharide-based hydrogel scaffold segment is expected to register a robust compound annual growth rate (CAGR) between 2025 and 2030. While precise figures vary, industry consensus points to a CAGR in the high single digits to low double digits, reflecting expanding clinical applications, regulatory approvals, and increased investment in regenerative medicine. Major suppliers like Cytiva (formerly GE Healthcare Life Sciences) and Lonza Group are scaling up production capacities and offering GMP-grade hydrogels to meet anticipated demand from both research and therapeutic markets.
Looking ahead, the next few years are likely to see further convergence of digital manufacturing, personalized medicine, and advanced biomaterials. Strategic partnerships between biomaterials companies, bioprinting technology providers, and healthcare institutions will be pivotal in translating laboratory breakthroughs into clinical therapies. As regulatory pathways for advanced tissue products become clearer, polysaccharide-based hydrogel scaffolds are poised to play a central role in the evolution of tissue engineering and regenerative medicine.
Sources & References
- Thermo Fisher Scientific
- Evonik Industries
- DuPont
- CELLINK
- DSM
- GELITA
- Organovo Holdings
- Lifecore Biomedical
- International Organization for Standardization
- BASF
- Nippon Kayaku
- Thermo Fisher Scientific