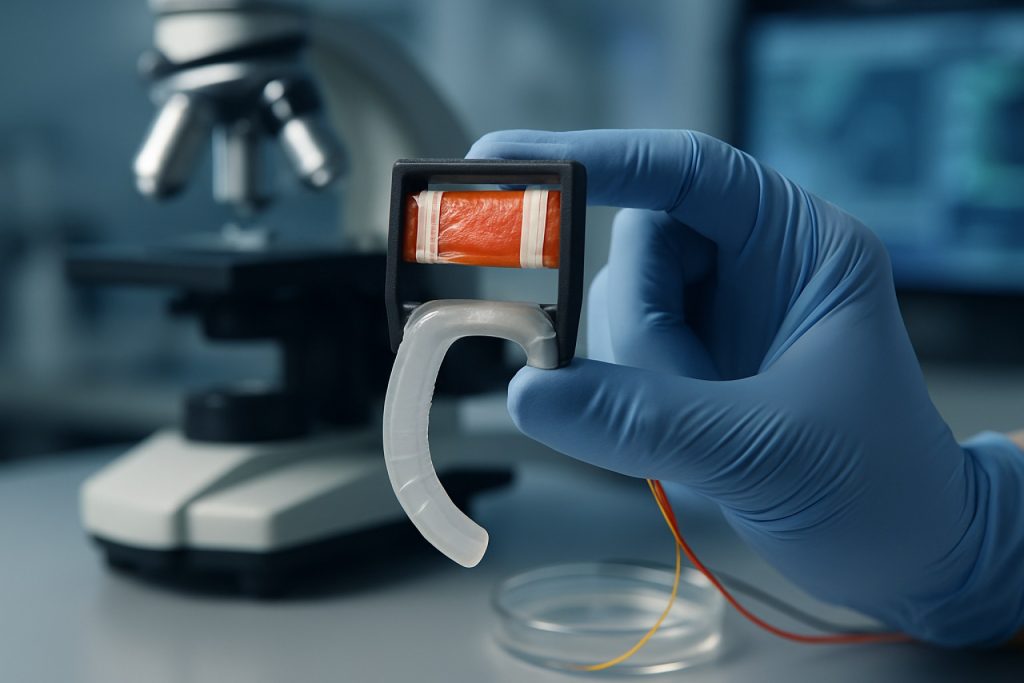
Biohybrid Actuator Engineering in 2025: How Living Materials Are Transforming Robotics and Medical Devices. Explore the Breakthroughs, Market Growth, and Future Disruptions Shaping This Cutting-Edge Sector.
- Executive Summary: Key Trends and Market Drivers in 2025
- Market Size and Growth Forecast (2025–2029): CAGR and Revenue Projections
- Core Technologies: Biomaterials, Cell Integration, and Actuation Mechanisms
- Leading Companies and Research Institutions (e.g., asme.org, ieee.org, mit.edu)
- Emerging Applications: Soft Robotics, Medical Devices, and Wearables
- Regulatory Landscape and Industry Standards (e.g., ieee.org, fda.gov)
- Investment Landscape: Funding, M&A, and Startup Activity
- Challenges: Scalability, Biocompatibility, and Manufacturing Bottlenecks
- Regional Analysis: North America, Europe, Asia-Pacific, and Rest of World
- Future Outlook: Disruptive Innovations and Strategic Roadmap to 2030
- Sources & References
Executive Summary: Key Trends and Market Drivers in 2025
Biohybrid actuator engineering, which integrates living biological tissues or cells with synthetic materials to create responsive, adaptive movement systems, is poised for significant advances in 2025. The field is driven by the convergence of tissue engineering, soft robotics, and advanced materials science, with applications spanning medical devices, soft robotics, and next-generation prosthetics. Key trends and market drivers in 2025 include the maturation of scalable manufacturing techniques, increased investment from both established industry players and startups, and a growing demand for bioinspired solutions in healthcare and automation.
A major trend is the transition from proof-of-concept laboratory demonstrations to early-stage commercial prototypes. Companies specializing in soft robotics and biomaterials, such as Boston Scientific and Medtronic, are exploring biohybrid actuators for minimally invasive surgical tools and implantable devices. These organizations are leveraging their expertise in biocompatible materials and device integration to accelerate the translation of biohybrid technologies into regulated medical markets.
In parallel, startups and research spinouts are focusing on the development of muscle-powered actuators and biohybrid soft robots for applications in drug delivery, micro-manipulation, and environmental sensing. For example, Myoware is advancing bioelectronic interfaces that harness living muscle tissue for actuation, while Soft Robotics Inc. is expanding its portfolio to include bioinspired gripping and manipulation systems. These efforts are supported by increasing venture capital interest and public-private partnerships, particularly in North America, Europe, and East Asia.
Material innovation remains a critical driver, with companies such as DSM and Evonik Industries supplying advanced polymers and hydrogels tailored for cell compatibility, mechanical resilience, and electrical responsiveness. The integration of living cells with synthetic scaffolds is being refined to enable longer operational lifespans and more robust performance under physiological conditions.
Looking ahead, the outlook for biohybrid actuator engineering in the next few years is marked by rapid prototyping, regulatory engagement, and the emergence of pilot manufacturing lines. As the sector moves toward clinical and industrial validation, collaborations between device manufacturers, biomaterials suppliers, and robotics firms are expected to intensify. The continued evolution of standards and best practices, led by industry bodies and regulatory agencies, will further shape the trajectory of this transformative field.
Market Size and Growth Forecast (2025–2029): CAGR and Revenue Projections
Biohybrid actuator engineering, which integrates living cells or tissues with synthetic materials to create responsive, adaptive movement systems, is emerging as a transformative segment within the broader soft robotics and biomaterials markets. As of 2025, the global market for biohybrid actuators remains in its nascent stage, but is projected to experience robust growth over the next five years, driven by advances in tissue engineering, microfabrication, and the increasing demand for soft, biocompatible robotic systems in medical, research, and industrial applications.
Current estimates suggest that the biohybrid actuator market is valued at under $100 million in 2025, reflecting its early commercialization phase. However, the sector is expected to achieve a compound annual growth rate (CAGR) exceeding 30% through 2029, with revenue projections reaching between $350 million and $500 million by the end of the forecast period. This rapid expansion is underpinned by several converging trends: the miniaturization of bioactuators for micro-robotics, the development of advanced cell culture and scaffold technologies, and the growing interest in bioinspired robotics for minimally invasive surgery, drug delivery, and environmental sensing.
Key industry players are beginning to scale up their research and development efforts. For example, Takeda Pharmaceutical Company has invested in biohybrid tissue platforms for regenerative medicine, while ABB and Boston Dynamics are exploring soft robotics and actuator technologies that could integrate biohybrid components in the future. In the academic-to-commercial pipeline, spinouts from leading institutions such as the Wyss Institute at Harvard and the Max Planck Institute for Intelligent Systems are actively developing biohybrid actuator prototypes, with some moving toward pilot-scale manufacturing.
Geographically, North America and Europe are expected to dominate early market growth due to strong research ecosystems and supportive regulatory environments. However, significant investments in biotechnology and robotics in East Asia—particularly in Japan and South Korea—are likely to accelerate regional adoption and innovation.
Looking ahead, the market outlook for biohybrid actuator engineering is highly positive, with anticipated breakthroughs in cell-material interfaces, scalable manufacturing, and application-specific customization. As regulatory pathways for biohybrid medical devices become clearer and industry standards emerge, the sector is poised for accelerated commercialization and broader adoption across healthcare, research, and industrial automation by 2029.
Core Technologies: Biomaterials, Cell Integration, and Actuation Mechanisms
Biohybrid actuator engineering, at the intersection of biology and robotics, is rapidly advancing as researchers and companies leverage living cells and engineered biomaterials to create soft, adaptive actuators. The core technologies underpinning this field are biomaterials selection, cell integration strategies, and the development of actuation mechanisms that harness biological processes for mechanical output.
In 2025, the focus remains on optimizing biomaterials that are both biocompatible and mechanically robust. Hydrogels, such as those based on collagen, gelatin, and alginate, are widely used due to their tunable stiffness and ability to support cell viability. Companies like Cytiva (formerly GE Healthcare Life Sciences) and Sigma-Aldrich (a subsidiary of Merck KGaA) supply a range of high-purity biomaterials tailored for tissue engineering and actuator fabrication. These materials are being engineered to better mimic the extracellular matrix, improving integration with living cells and enhancing actuator performance.
Cell integration is another critical area, with muscle cells (myocytes) from animal or human sources being the most common actuators due to their contractile properties. Recent advances in stem cell differentiation and genetic engineering have enabled the production of more robust and responsive muscle tissues. Companies such as Lonza and Thermo Fisher Scientific provide primary cells and stem cell lines, as well as reagents for cell culture and differentiation, supporting the development of biohybrid actuators with improved longevity and force output.
Actuation mechanisms in biohybrid systems typically rely on electrical or optical stimulation to induce contraction in muscle-based actuators. In 2025, there is a growing trend toward integrating microelectrode arrays and optogenetic tools for precise, programmable control. Companies like Multi Channel Systems are supplying advanced stimulation and recording platforms that facilitate the development and testing of these biohybrid devices.
Looking ahead, the next few years are expected to see further convergence of synthetic and biological components, with the integration of nanoscale sensors and wireless control systems. The development of scalable manufacturing processes for biohybrid actuators is also a key focus, as companies and research groups aim to transition from proof-of-concept prototypes to commercially viable products for applications in soft robotics, medical devices, and drug testing platforms. As the field matures, collaborations between biomaterials suppliers, cell technology companies, and device manufacturers will be crucial in overcoming current limitations and unlocking the full potential of biohybrid actuator engineering.
Leading Companies and Research Institutions (e.g., asme.org, ieee.org, mit.edu)
Biohybrid actuator engineering—a field at the intersection of biology, materials science, and robotics—has seen significant momentum in 2025, with leading companies and research institutions driving innovation. These actuators, which integrate living cells or tissues with synthetic frameworks, are enabling new classes of soft robots and medical devices with unprecedented adaptability and responsiveness.
Among the most prominent research institutions, the Massachusetts Institute of Technology (MIT) continues to be a global leader. MIT’s Department of Mechanical Engineering and its affiliated labs have published several high-impact studies in 2024–2025, demonstrating muscle-cell-powered micro-actuators and biohybrid swimmers capable of complex locomotion. Their work often leverages advanced microfabrication and tissue engineering techniques, setting benchmarks for performance and scalability.
In Europe, the ETH Zurich has established itself as a powerhouse in biohybrid robotics. ETH’s Institute of Robotics and Intelligent Systems has developed biohybrid actuators using engineered skeletal muscle tissues, focusing on applications in soft robotics and biomedical devices. Their collaborations with industry partners are accelerating the translation of lab-scale prototypes to functional demonstrators.
Japan’s RIKEN research institute is also at the forefront, with its Bioengineering Laboratory pioneering the integration of living muscle fibers into micro-robotic systems. RIKEN’s recent breakthroughs include the development of biohybrid actuators with improved longevity and force output, addressing key challenges in the field.
On the corporate side, TDK Corporation—a major Japanese electronics manufacturer—has expanded its advanced materials division to include biohybrid actuator components. TDK’s expertise in piezoelectric and soft electronic materials is being leveraged to create hybrid systems that combine living cells with responsive polymers, targeting next-generation medical devices and wearable robotics.
In the United States, Abbott Laboratories is exploring biohybrid actuator technologies for minimally invasive surgical tools and implantable devices. Their R&D teams are collaborating with academic partners to integrate living tissues into device platforms, aiming for enhanced biocompatibility and functional integration.
Industry bodies such as the American Society of Mechanical Engineers (ASME) and the Institute of Electrical and Electronics Engineers (IEEE) are playing a crucial role by organizing conferences, publishing standards, and fostering interdisciplinary collaboration. Their 2025 events have featured dedicated tracks on biohybrid actuators, reflecting the sector’s rapid maturation.
Looking ahead, the synergy between leading research institutions and forward-thinking companies is expected to accelerate commercialization. With ongoing advances in cell engineering, materials science, and device integration, biohybrid actuator engineering is poised for breakthroughs in medical, industrial, and consumer robotics over the next few years.
Emerging Applications: Soft Robotics, Medical Devices, and Wearables
Biohybrid actuator engineering, which integrates living cells or tissues with synthetic materials to create responsive, adaptive systems, is rapidly advancing in 2025, with significant implications for soft robotics, medical devices, and wearable technologies. The field is characterized by the convergence of tissue engineering, materials science, and robotics, enabling the development of actuators that mimic or surpass the capabilities of natural muscles.
In soft robotics, biohybrid actuators are being leveraged to create robots with unprecedented flexibility and adaptability. Companies such as SoftBank Robotics and Boston Dynamics are exploring the integration of biohybrid components to enhance the dexterity and lifelike movement of their robotic platforms. While these firms are renowned for their advanced robotics, collaborations with academic and biotech partners are driving the incorporation of living muscle tissues into robotic actuators, aiming for robots that can perform delicate tasks in unstructured environments.
In the medical device sector, biohybrid actuators are being developed for next-generation prosthetics and implantable devices. For example, Medtronic and Smith+Nephew are investing in research to create biohybrid-driven prosthetic limbs and cardiac assist devices that offer more natural movement and improved biocompatibility. These actuators, often composed of engineered muscle cells on biocompatible scaffolds, can respond to electrical or chemical stimuli, closely mimicking the function of native tissues. Early-stage clinical trials are anticipated within the next few years, focusing on safety, integration, and long-term performance.
Wearable technology is another area witnessing rapid adoption of biohybrid actuator engineering. Companies like Owlet and Fitbit are monitoring advances in soft, biohybrid actuators for potential use in adaptive wearables that can conform to the body, provide haptic feedback, or assist with movement. The integration of living cells allows for devices that are not only more comfortable but also capable of dynamic responses to physiological changes, opening new possibilities for personalized health monitoring and rehabilitation.
Looking ahead, the outlook for biohybrid actuator engineering is highly promising. The next few years are expected to see the transition from laboratory prototypes to commercial products, driven by advances in cell engineering, scalable manufacturing, and regulatory approvals. As industry leaders and startups continue to collaborate, the emergence of biohybrid actuators in soft robotics, medical devices, and wearables is poised to redefine the boundaries of human-machine interaction and biomedical innovation.
Regulatory Landscape and Industry Standards (e.g., ieee.org, fda.gov)
The regulatory landscape for biohybrid actuator engineering is rapidly evolving as these technologies transition from laboratory prototypes to potential commercial and clinical applications. Biohybrid actuators—devices that integrate living cells or tissues with synthetic materials to produce movement—pose unique challenges for regulators due to their hybrid biological and mechanical nature. As of 2025, regulatory frameworks are being shaped by both medical device and biotechnology standards, with a focus on safety, efficacy, and ethical considerations.
In the United States, the U.S. Food and Drug Administration (FDA) is the primary authority overseeing the approval of biohybrid actuators intended for medical use, such as soft robotic prosthetics or implantable devices. The FDA classifies these products under its medical device regulations, but the presence of living cells may also trigger oversight under biologics regulations. The agency has issued guidance on combination products and regenerative medicine, which are relevant to biohybrid actuators, and is expected to release more targeted guidelines as the field matures. The FDA’s Center for Devices and Radiological Health (CDRH) is actively engaging with industry and academic stakeholders to clarify premarket requirements, including biocompatibility, sterility, and long-term performance testing.
Globally, regulatory bodies such as the European Medicines Agency (EMA) and the Medical Device Coordination Group (MDCG) under the European Union’s Medical Device Regulation (MDR) are also adapting their frameworks. The MDR, which came into full effect in 2021, emphasizes risk management, clinical evaluation, and post-market surveillance for innovative devices, including those with biological components. The EMA is collaborating with international partners to harmonize standards for advanced therapy medicinal products (ATMPs), which may encompass certain biohybrid actuators.
Industry standards are being developed in parallel by organizations such as the IEEE and the International Organization for Standardization (ISO). The IEEE has established working groups focused on soft robotics and biohybrid systems, aiming to define terminology, performance metrics, and safety protocols. ISO’s Technical Committee 150 (Implants for surgery) and Technical Committee 299 (Robotics) are both exploring standards relevant to biohybrid actuators, particularly regarding material compatibility and functional testing.
Looking ahead, the next few years are expected to see increased collaboration between regulatory agencies, standards bodies, and industry leaders to address the unique challenges of biohybrid actuator engineering. As clinical trials and commercial deployments expand, regulators will likely refine requirements for preclinical testing, manufacturing quality, and post-market monitoring. Companies at the forefront of biohybrid actuator development are anticipated to play a key role in shaping these standards through active participation in regulatory consultations and standards committees.
Investment Landscape: Funding, M&A, and Startup Activity
The investment landscape for biohybrid actuator engineering in 2025 is characterized by a surge in early-stage funding, strategic partnerships, and a growing number of startups aiming to commercialize breakthroughs in soft robotics and living-machine interfaces. Biohybrid actuators—devices that integrate living cells or tissues with synthetic materials to produce movement—are attracting attention from both established robotics firms and venture capitalists, driven by their potential in medical devices, soft robotics, and adaptive prosthetics.
In the past year, several startups have secured seed and Series A rounds to advance biohybrid actuator technologies. Notably, companies such as Cyfuse Biomedical (Japan) and TISSIUM (France) have expanded their R&D efforts into biohybrid systems, leveraging their expertise in bioprinting and tissue engineering. Cyfuse Biomedical is recognized for its Kenzan method of 3D bioprinting, which is being adapted for the fabrication of muscle-based actuators. Meanwhile, TISSIUM has announced collaborations with academic labs to explore biohybrid soft robotics for minimally invasive surgery.
On the corporate side, established robotics and automation companies are increasingly investing in or acquiring startups with biohybrid capabilities. ABB, a global leader in robotics, has signaled interest in soft robotics and biohybrid actuation through its innovation arms and open innovation challenges. Similarly, Boston Scientific has initiated partnerships with university spinouts to explore biohybrid actuators for next-generation implantable devices.
The sector is also witnessing the emergence of university spinouts, particularly from institutions with strong bioengineering and robotics programs. For example, spinouts from ETH Zurich and MIT are developing muscle-powered micro-actuators for drug delivery and micro-manipulation, attracting grants and angel investment. These startups are often supported by technology transfer offices and incubators, which facilitate connections with industry partners and investors.
Mergers and acquisitions remain limited but are expected to increase as the technology matures and regulatory pathways become clearer. The next few years are likely to see more cross-sector deals, especially as medical device manufacturers seek to integrate biohybrid actuators into their product pipelines. The outlook for 2025 and beyond is optimistic, with continued growth in funding, increased corporate engagement, and a steady influx of new entrants aiming to bridge the gap between laboratory innovation and commercial application.
Challenges: Scalability, Biocompatibility, and Manufacturing Bottlenecks
Biohybrid actuator engineering, which integrates living cells or tissues with synthetic materials to create responsive, adaptive systems, faces several critical challenges as the field moves into 2025 and beyond. The most pressing issues are scalability, biocompatibility, and manufacturing bottlenecks, each of which must be addressed to enable commercial and clinical translation.
Scalability remains a significant hurdle. While proof-of-concept devices—such as muscle-powered micro-robots and soft grippers—have been demonstrated in academic settings, scaling these systems for industrial or medical applications is complex. The reproducible cultivation of contractile cells (e.g., myocytes) at large volumes, and their integration with synthetic scaffolds, requires advanced bioprocessing infrastructure. Companies like Organovo and Aleph Farms have developed bioprinting and tissue engineering platforms, but these are primarily focused on tissue for regenerative medicine and food, not actuators. Adapting such platforms for actuator production will require new protocols for cell alignment, vascularization, and long-term viability.
Biocompatibility is another central challenge, especially for actuators intended for implantation or direct interaction with biological tissues. Synthetic scaffolds must support cell adhesion, proliferation, and function without eliciting immune responses or toxicity. Materials such as polydimethylsiloxane (PDMS) and hydrogels are commonly used, but their long-term biocompatibility and mechanical stability under physiological conditions are still under investigation. Companies like Corning Incorporated and Cytiva (formerly GE Life Sciences) supply advanced biomaterials and cell culture systems, yet the translation of these materials into robust, implantable actuators is ongoing. The development of new bioinspired polymers and hybrid composites is expected to accelerate in the next few years, driven by collaborations between material suppliers and bioengineering startups.
Manufacturing bottlenecks are particularly acute due to the need for precise spatial organization of living and non-living components. Current fabrication methods, such as 3D bioprinting and micro-molding, are limited by throughput, resolution, and the ability to maintain cell viability during processing. Automation and standardization are lacking, making it difficult to produce large batches of identical biohybrid actuators. Industry leaders in bioprinting hardware, such as CELLINK (now part of BICO Group), are working to improve printhead technology, multi-material integration, and real-time quality control. However, widespread adoption will depend on further advances in process monitoring, closed-loop control, and regulatory compliance.
Looking ahead, overcoming these challenges will require coordinated efforts across cell biology, materials science, and manufacturing engineering. The next few years are likely to see increased investment in scalable bioprocessing, the development of new biocompatible materials, and the automation of biohybrid actuator assembly, setting the stage for broader adoption in soft robotics, medical devices, and beyond.
Regional Analysis: North America, Europe, Asia-Pacific, and Rest of World
Biohybrid actuator engineering—a field at the intersection of biology, materials science, and robotics—has seen significant regional differentiation in research focus, commercialization, and infrastructure development as of 2025. The global landscape is shaped by the interplay of academic leadership, industrial investment, and regulatory environments across North America, Europe, Asia-Pacific, and the Rest of World.
North America remains a global leader, driven by robust academic research and early-stage commercialization. The United States, in particular, benefits from the convergence of top-tier universities and a dynamic startup ecosystem. Institutions such as MIT and Harvard have spun out ventures focused on muscle-cell-based actuators and soft robotics. Companies like Thermo Fisher Scientific and Cytiva supply critical cell culture and biomaterials platforms, supporting both research and pilot-scale manufacturing. The region’s regulatory agencies, such as the FDA, are actively engaging with the sector to develop frameworks for biohybrid medical devices, which is expected to accelerate clinical translation in the coming years.
Europe is characterized by strong public funding and cross-border collaborations. The European Union’s Horizon Europe program has allocated substantial grants to biohybrid robotics and actuator projects, fostering partnerships between universities, research institutes, and industry. Germany, the Netherlands, and Switzerland are particularly active, with organizations like Eppendorf and Sartorius providing advanced bioprocessing tools. The region’s emphasis on ethical standards and sustainability is shaping the development of biodegradable and environmentally friendly actuator systems. Regulatory harmonization across EU member states is expected to streamline market entry for biohybrid devices by 2027.
Asia-Pacific is rapidly expanding its footprint, with Japan, South Korea, and China investing heavily in biohybrid actuator R&D. Japanese firms such as Olympus Corporation and Fujifilm are leveraging their expertise in precision engineering and biomaterials to develop next-generation soft actuators for medical and industrial applications. China’s government-backed initiatives are fostering university-industry consortia, with a focus on scalable manufacturing and integration into robotics. The region is expected to see the fastest growth in commercial deployment, particularly in healthcare and assistive robotics, through 2028.
Rest of World regions, including Latin America and the Middle East, are at earlier stages but show growing interest. Collaborations with North American and European institutions are helping to build local capacity. Access to advanced biomanufacturing equipment from global suppliers is gradually enabling pilot projects, especially in academic settings.
Overall, the next few years will likely see North America and Europe maintaining leadership in innovation and regulatory frameworks, while Asia-Pacific drives rapid commercialization and scale-up. Cross-regional partnerships and harmonized standards are anticipated to accelerate the global adoption of biohybrid actuator technologies.
Future Outlook: Disruptive Innovations and Strategic Roadmap to 2030
Biohybrid actuator engineering, which integrates living cells or tissues with synthetic materials to create responsive, adaptive movement, is poised for significant advances through 2025 and into the next decade. The field is rapidly evolving from proof-of-concept demonstrations to early-stage commercial and translational applications, driven by breakthroughs in cell engineering, soft robotics, and materials science.
In 2025, the most notable progress is expected in the development of scalable, robust biohybrid actuators for soft robotics and biomedical devices. Companies such as Tessera Therapeutics and Organovo Holdings, Inc.—both recognized for their expertise in synthetic biology and tissue engineering—are actively exploring the integration of engineered muscle tissues with flexible substrates. These efforts aim to create actuators that mimic natural muscle contraction, offering unprecedented compliance and adaptability for next-generation medical devices and soft robotic systems.
Academic-industry collaborations are accelerating the translation of laboratory advances into practical devices. For example, partnerships between research institutions and companies like CELLINK (a leader in bioprinting and biofabrication) are focusing on the scalable production of living muscle fibers and their assembly into functional actuator units. This approach is expected to address key challenges such as long-term viability, force output, and integration with electronic control systems.
Looking ahead to 2030, the strategic roadmap for biohybrid actuator engineering includes several disruptive innovations:
- Autonomous Biohybrid Systems: The convergence of optogenetics, synthetic biology, and microfluidics is anticipated to yield actuators capable of self-regulation and adaptive behavior, with companies like Emulate, Inc. (noted for organ-on-chip platforms) positioned to leverage their expertise in microenvironment control.
- Implantable and Wearable Devices: Biohybrid actuators are expected to enable new classes of implantable medical devices—such as artificial sphincters or cardiac assist devices—where biocompatibility and dynamic responsiveness are critical. Firms specializing in medical device innovation, such as Medtronic, are likely to play a role in clinical translation.
- Environmental and Industrial Robotics: The unique adaptability of biohybrid actuators may be harnessed for soft robots operating in unstructured environments, with potential applications in search-and-rescue, environmental monitoring, and precision agriculture.
By 2030, the integration of living and synthetic components is projected to move beyond niche applications, with standardized manufacturing protocols and regulatory frameworks emerging. The sector’s trajectory will be shaped by ongoing advances in cell sourcing, bioprinting, and smart material interfaces, as well as by the strategic investments of leading bioengineering and robotics companies.
Sources & References
- Boston Scientific
- Medtronic
- Myoware
- Soft Robotics Inc.
- DSM
- Evonik Industries
- Takeda Pharmaceutical Company
- Boston Dynamics
- Thermo Fisher Scientific
- Multi Channel Systems
- Massachusetts Institute of Technology
- ETH Zurich
- RIKEN
- American Society of Mechanical Engineers
- Institute of Electrical and Electronics Engineers
- SoftBank Robotics
- Smith+Nephew
- Fitbit
- Cyfuse Biomedical
- TISSIUM
- Organovo
- Aleph Farms
- CELLINK
- Eppendorf
- Sartorius
- Olympus Corporation
- Fujifilm
- Emulate, Inc.